Amino Acids, Proteins & Protein Structure (Edexcel International A Level (IAL) Biology) : Revision Note
Amino Acids Structure
Proteins
Proteins are polymers (and macromolecules) made of monomers called amino acids
The sequence, type and number of the amino acids within a protein determines its shape and therefore its function
Proteins are extremely important in cells because they form all of the following:
Enzymes
Cell membrane proteins (eg. carrier)
Hormones
Immunoproteins (eg. immunoglobulins)
Transport proteins (eg. haemoglobin)
Structural proteins (eg. keratin, collagen)
Contractile proteins (eg. myosin)
Amino acids
Amino acids are the monomers of polypeptides
There are 20 amino acids found in proteins common to all living organisms
The general structure of all amino acids is a central carbon atom bonded to:
An amine (also called amino) group -NH2
A carboxylic acid group -COOH
A hydrogen atom
An R group (which is how each amino acid differs and why amino acid properties differ e.g. whether they are acidic or basic or whether they are polar or non-polar)
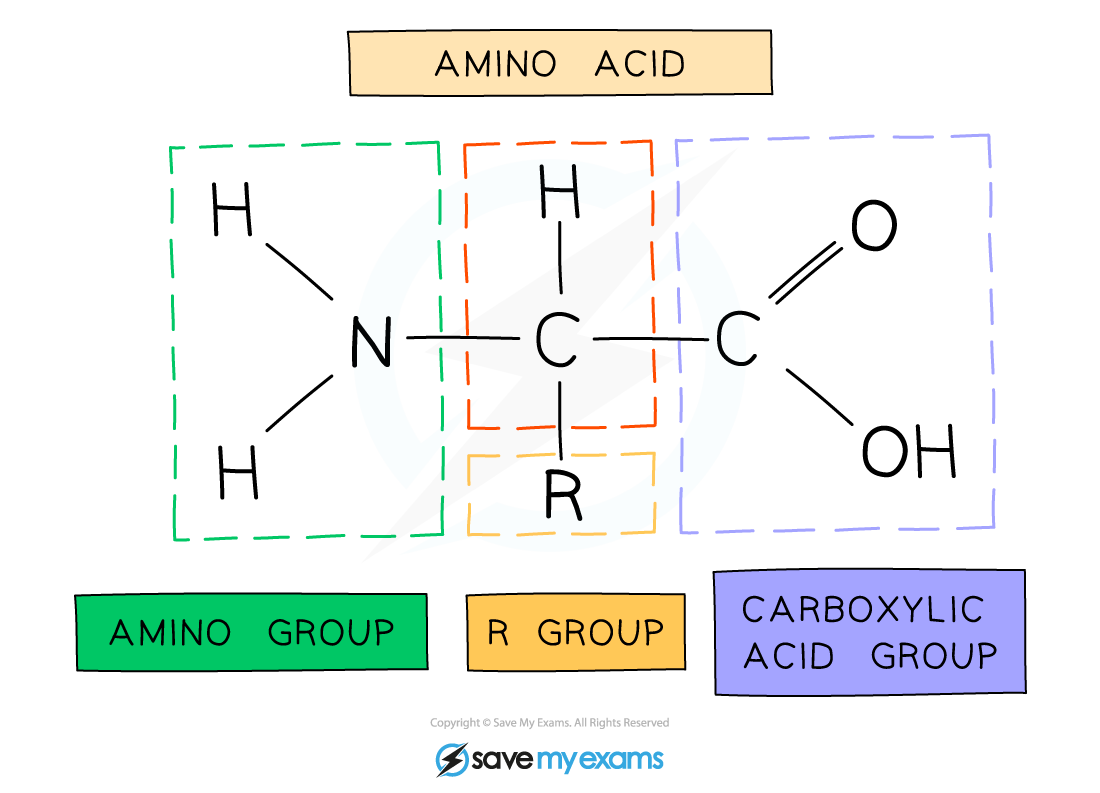
The general structure of an amino acid
Polypeptides & Protein Structure
Peptide bonds form between amino acids
Peptide bonds are covalent bonds and so involve the sharing of electrons
In order to form a peptide bond :
A hydroxyl (-OH) is lost from the carboxylic group of one amino acid
A hydrogen atom is lost from the amine group of another amino acid
The remaining carbon atom (with the double-bonded oxygen) from the first amino acid bonds to the nitrogen atom of the second amino acid
This is a condensation reaction so water is released
Dipeptides are formed by the condensation of two amino acids
Polypeptides are formed by the condensation of many (3 or more) amino acids
A protein may have only one polypeptide chain or it may have multiple chains interacting with each other
During hydrolysis reactions, the addition of water breaks the peptide bonds resulting in polypeptides being broken down to amino acids
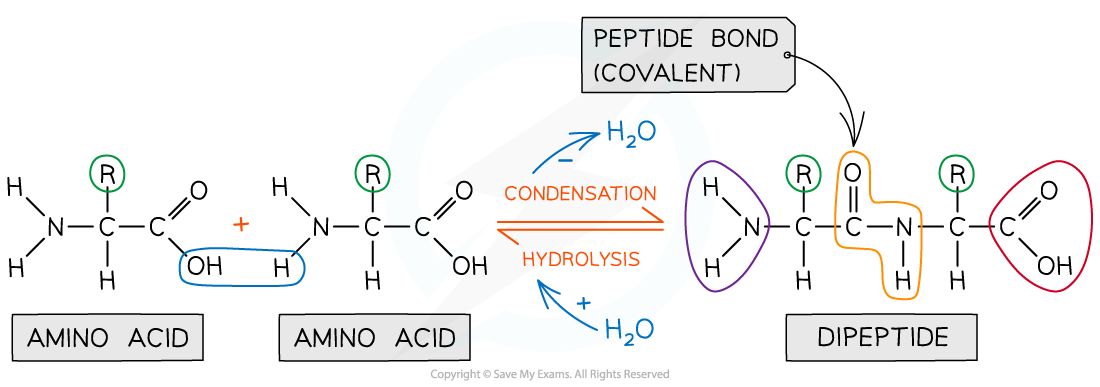
Peptide bonds are formed by condensation reactions (releasing a molecule of water) and broken by hydrolysis reactions (adding a molecule of water)
Examiner Tips and Tricks
When asked to identify the location of the peptide bond, look for where nitrogen is bonded to a carbon which has a double bond with an oxygen atom, note the R group is not involved in the formation of a peptide bond.
Structures of specific amino acids are not required.
Primary, Secondary & 3-D Structure of Proteins
There are four levels of structure in proteins
Three are related to a single polypeptide chain
The fourth level relates to a protein that has two or more polypeptide chains
Polypeptide or protein molecules can have anywhere from 3 amino acids (Glutathione) to more than 34,000 amino acids (Titin) bonded together in chains
Primary structure
The sequence of amino acids bonded by covalent peptide bonds is the primary structure of a protein
The DNA of a cell determines the primary structure of a protein by instructing the cell to add certain amino acids in specific quantities in a certain sequence. This affects the shape and therefore the function of the protein
The primary structure is specific for each protein (one alteration in the sequence of amino acids can affect the function of the protein)
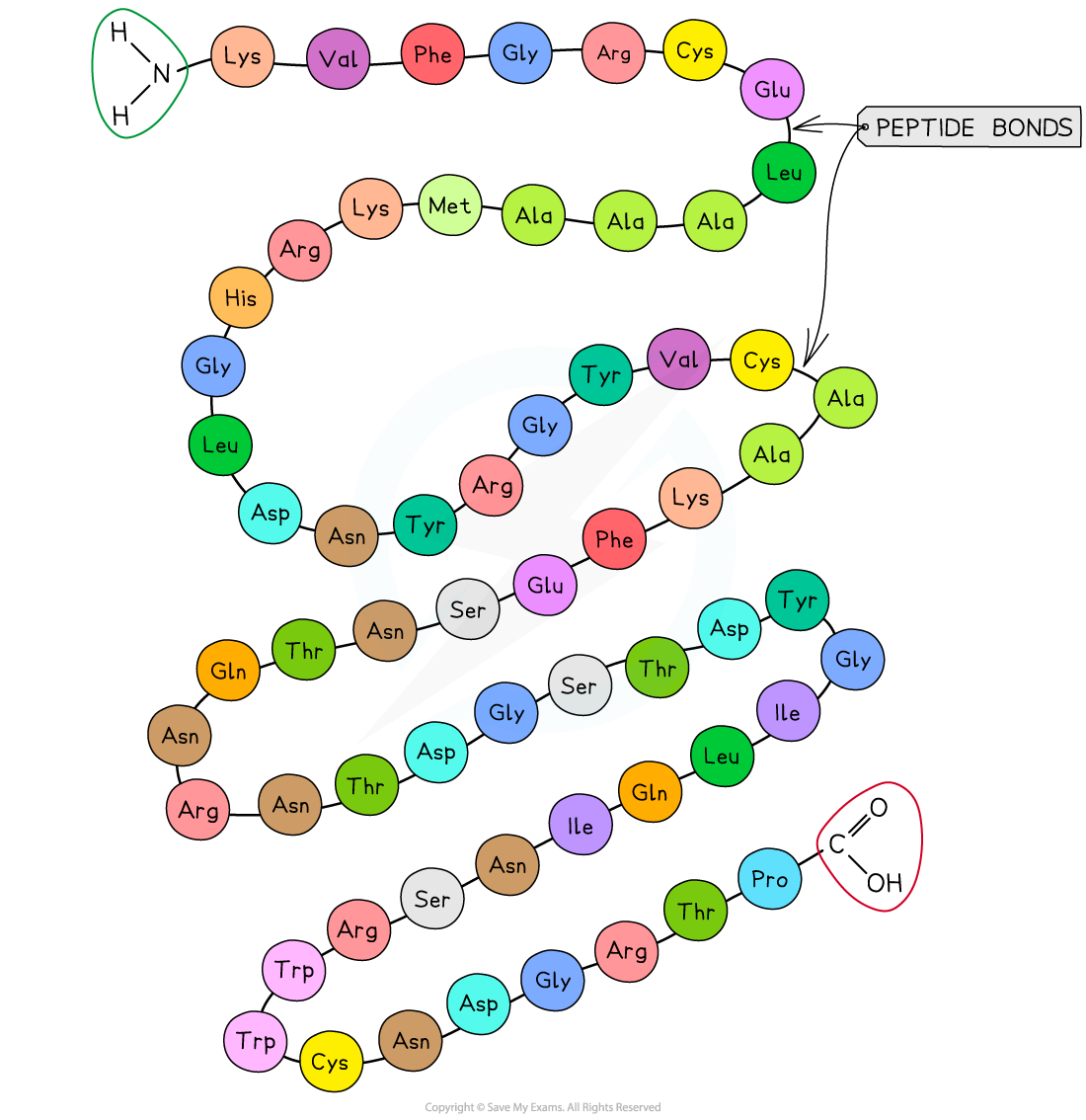
An example of the primary structure of proteins showing the three-letter abbreviation of specific amino acids
Secondary structure
The secondary structure of a protein occurs when the weak negatively charged nitrogen and oxygen atoms interact with the weak positively charged hydrogen atoms to form hydrogen bonds
There are two shapes that can form within proteins due to the hydrogen bonds:
α-helix
β-pleated sheet
The α-helix shape occurs when the hydrogen bonds form between every fourth peptide bond (between the oxygen of the carboxyl group and the hydrogen of the amine group)
The β-pleated sheet shape forms when the protein folds so that two parts of the polypeptide chain are parallel to each other enabling hydrogen bonds to form between parallel peptide bonds
Most fibrous proteins have secondary structures (e.g. collagen and keratin)
The secondary structure only relates to hydrogen bonds forming between the amino group and the carboxyl group (the ‘protein backbone’)
The hydrogen bonds can be broken by high temperatures and pH changes
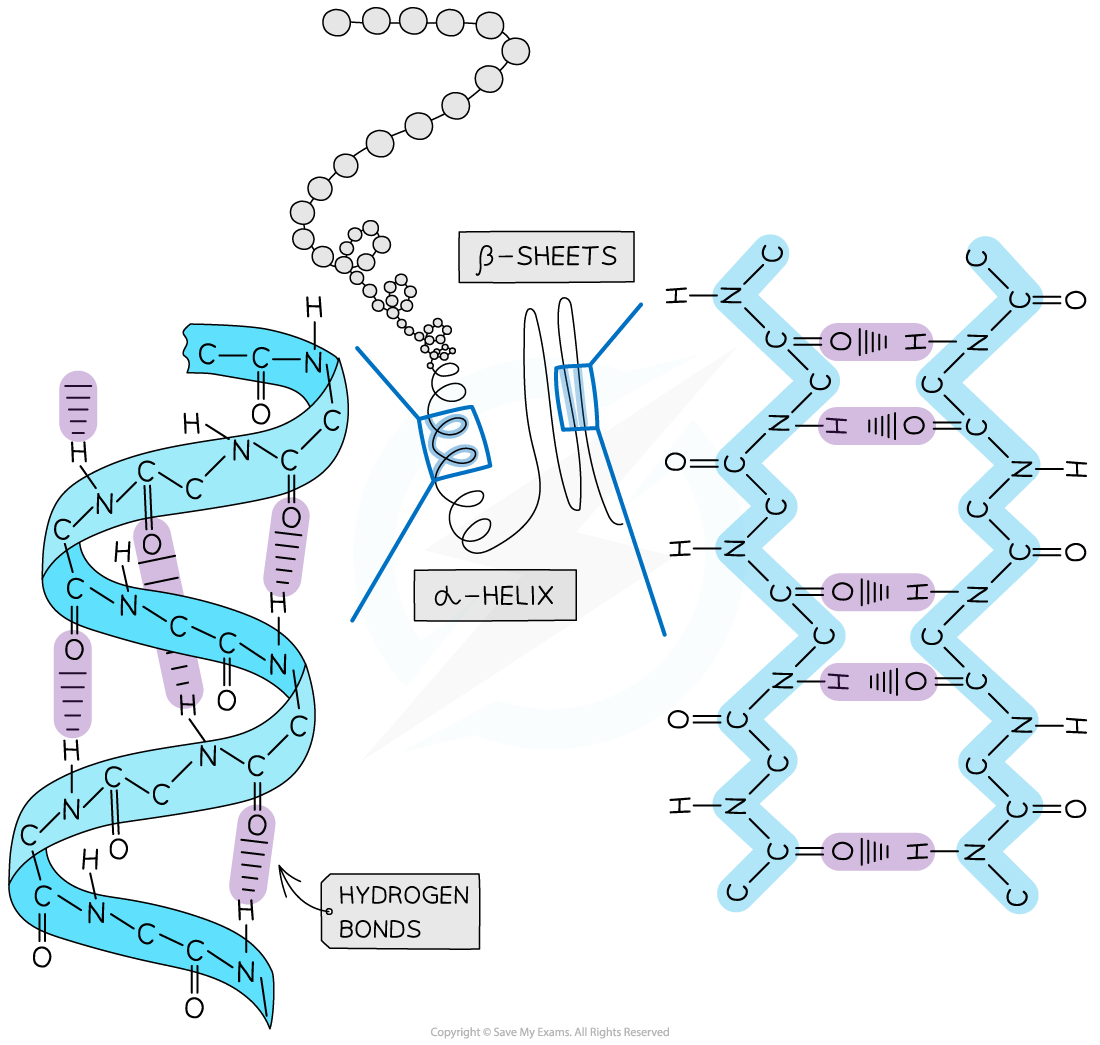
The secondary structure of a protein with the α-helix and β-pleated sheet shapes highlighted. The magnified regions illustrate how the hydrogen bonds form between the peptide bonds
Tertiary structure
Further conformational change of the secondary structure leads to additional bonds forming between the R groups (side chains)
The additional bonds are:
Hydrogen (these are between R groups)
Disulphide (only occurs between cysteine amino acids)
Ionic (occurs between charged R groups)
Weak hydrophobic interactions (between non-polar R groups)
This structure is common in 3D globular proteins
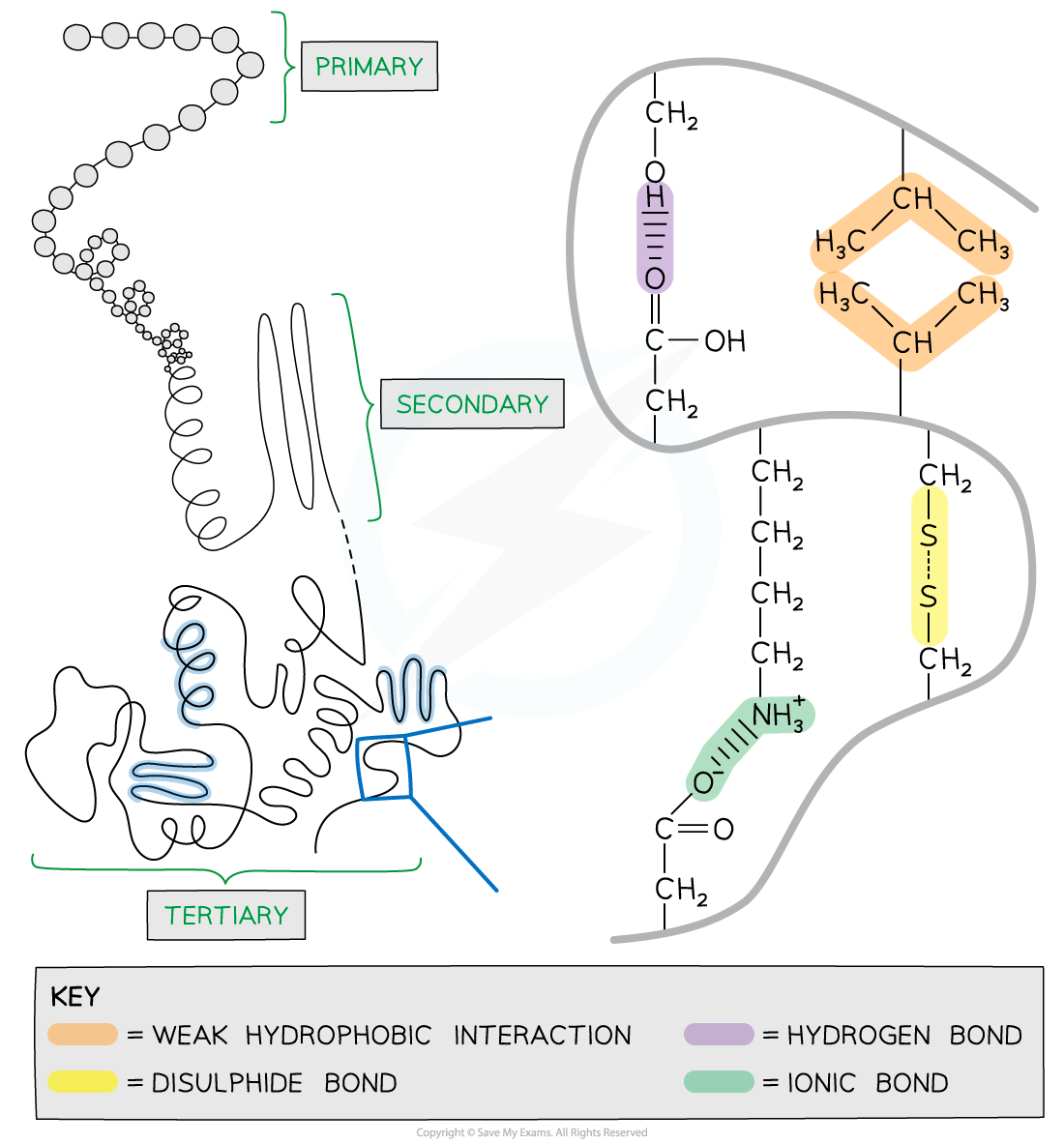
The 3D tertiary structure of proteins with hydrogen bonds, ionic bonds, disulphide bonds and hydrophobic interactions formed between the R groups of the amino acids
Quaternary structure
Occurs in proteins that have more than one polypeptide chain working together as a functional macromolecule, for example, haemoglobin
The same bonds responsible for maintaining the tertiary structure of a protein will also be involved in forming the quaternary structure
Each polypeptide chain in the quaternary structure is referred to as a subunit of the protein
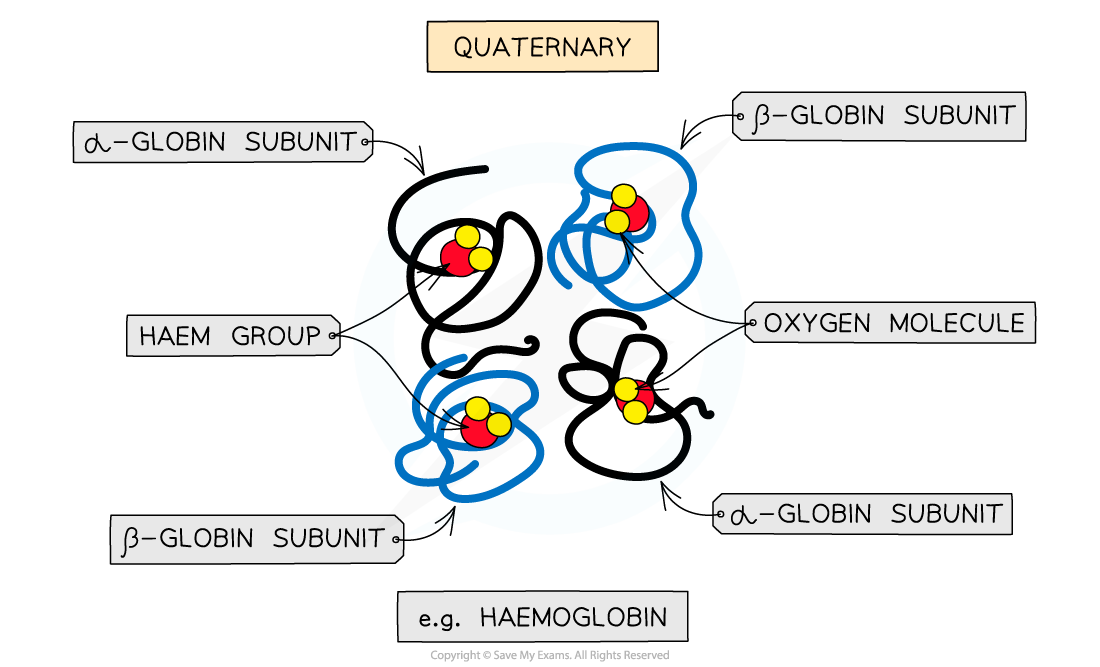
The quaternary structure of a protein. This is an example of haemoglobin which contains four subunits (polypeptide chains) working together to carry oxygen
Summary of Bonds in Proteins Table
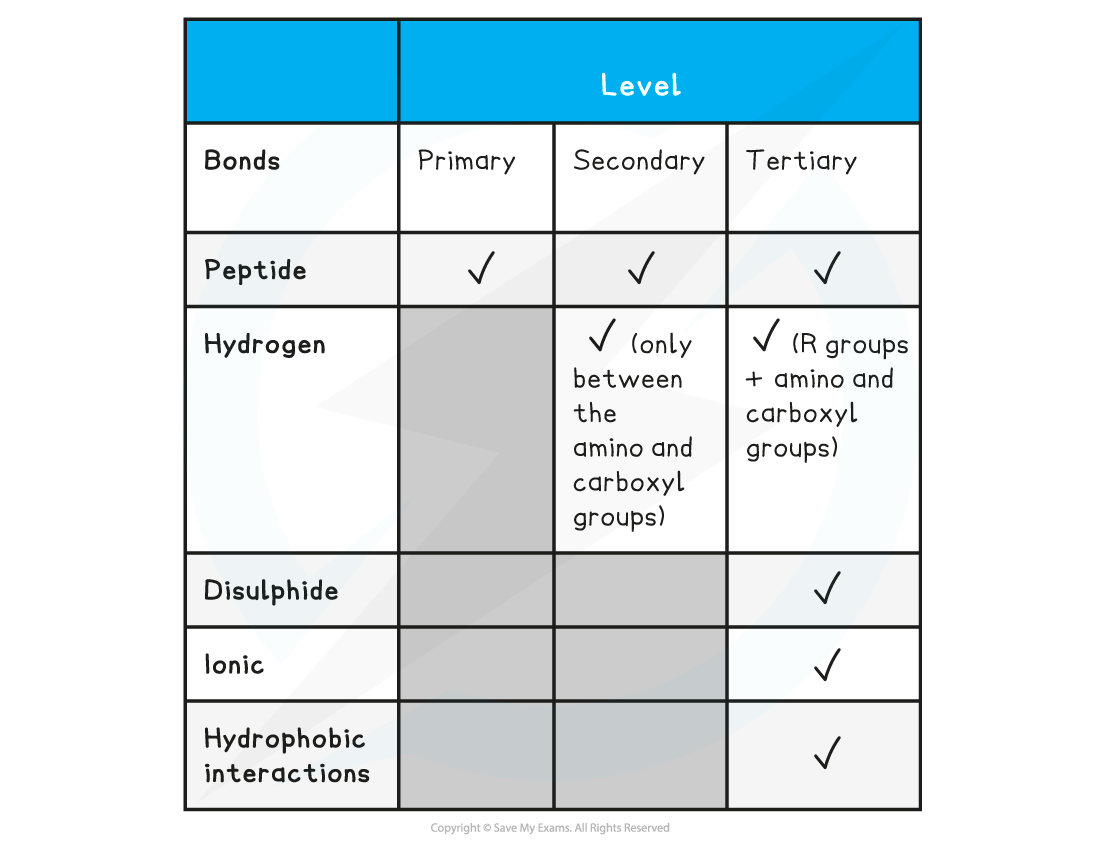
Examiner Tips and Tricks
Familiarise yourself with the difference between the four structural levels found in proteins, noting which bonds are found at which level. Remember that the hydrogen bonds in tertiary structures are between the R groups whereas in secondary structures the hydrogen bonds form between the amino and carboxyl groups.
Globular & Fibrous Proteins
Globular proteins: Structure
Globular proteins are
Compact
Roughly spherical (circular) in shape
Globular proteins form a spherical shape when folding into their tertiary structure because:
Their non-polar hydrophobic R groups are orientated towards the centre of the protein away from the aqueous surroundings
Their polar hydrophilic R groups orientate themselves on the outside of the protein
The folding of the protein due to the interactions between the R groups results in globular proteins having specific shapes
Some globular proteins are conjugated protein that contain a prosthetic group
Globular proteins: Function
The orientation of their R groups enables globular proteins to be (generally) soluble in water as the water molecules can surround the polar hydrophilic R groups
The solubility of globular proteins in water means they play important physiological roles as they can be easily transported around organisms and be involved in metabolic reactions
For example, enzymes can catalyse specific reactions and immunoglobulins can respond to specific antigens
Haemoglobin
Haemoglobin is a globular protein which is an oxygen-carrying pigment found in vast quantities in red blood cells
It has a quaternary structure as there are four polypeptide chains
These chains or subunits are globin proteins (two α–globins and two β–globins) and each subunit has a prosthetic haem group
The four globin subunits are held together by disulphide bonds
Their hydrophobic R groups are facing inwards (helping preserve the three-dimensional spherical shape)
Their hydrophilic R groups are facing outwards (helping maintain its solubility)
The arrangements of the R groups is important to the functioning of haemoglobin
If changes occur to the sequence of amino acids in the subunits this can result in the properties of haemoglobin changing
This is what happens to cause sickle cell anaemia (where base substitution results in the amino acid valine (non-polar) replacing glutamic acid (polar) making haemoglobin less soluble)
The prosthetic haem group contains an iron II ion (Fe2+) which is able to reversibly combine with an oxygen molecule forming oxyhaemoglobin and results in the haemoglobin appearing bright red
Each haemoglobin with the four haem groups can therefore carry four oxygen molecules (eight oxygen atoms)
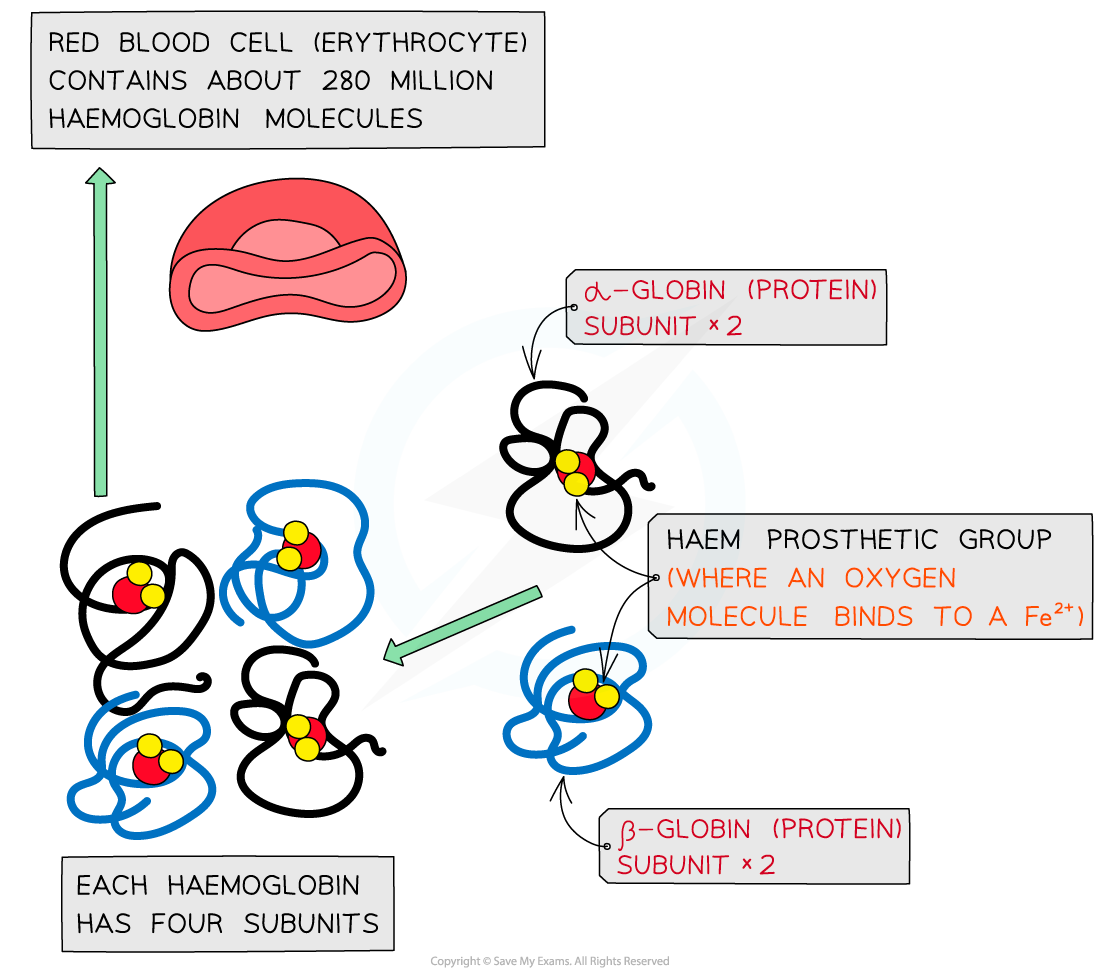
The molecular structure of haemoglobin showing the α-globin and β-globin subunits, the prosthetic haem group with oxygen molecules bonded to form oxyhaemoglobin
Haemoglobin is responsible for binding oxygen in the lungs and transporting the oxygen to tissue to be used in aerobic metabolic pathways
As oxygen is not very soluble in water and haemoglobin is, oxygen can be carried more efficiently around the body when bound to the haemoglobin
The presence of the haem group (and Fe2+) enables small molecules like oxygen to be bound more easily because as each oxygen molecule binds, it alters the quaternary structure (due to alterations in the tertiary structure) of the protein which causes haemoglobin to have a higher affinity for the subsequent oxygen molecules and they bind more easily
The existence of the iron II ion (Fe2+) in the prosthetic haem group also allows oxygen to reversibly bind as none of the amino acids that make up the polypeptide chains in haemoglobin are well suited to binding with oxygen
Fibrous proteins: Structure
Fibrous proteins are long strands of polypeptide chains that have cross-linkages due to hydrogen bonds
These proteins have little or no tertiary structure
Fibrous proteins have a limited number of amino acids with the sequence usually being highly repetitive
The highly repetitive sequence creates very organised structures
Fibrous proteins: Function
Due to a large number of hydrophobic R groups, fibrous proteins are insoluble in water
Fibrous proteins are strong and this, along with their insolubility property, makes fibrous proteins very suitable for structural roles
Examples of fibrous proteins:
Keratin makes up hair, nails, horns and feathers (it is a very tough fibrous protein)
Elastin is found in connective tissue, tendons, skin and bone (it can stretch and then return to its original shape)
Collagen is a connective tissue found in skin, tendons and ligaments
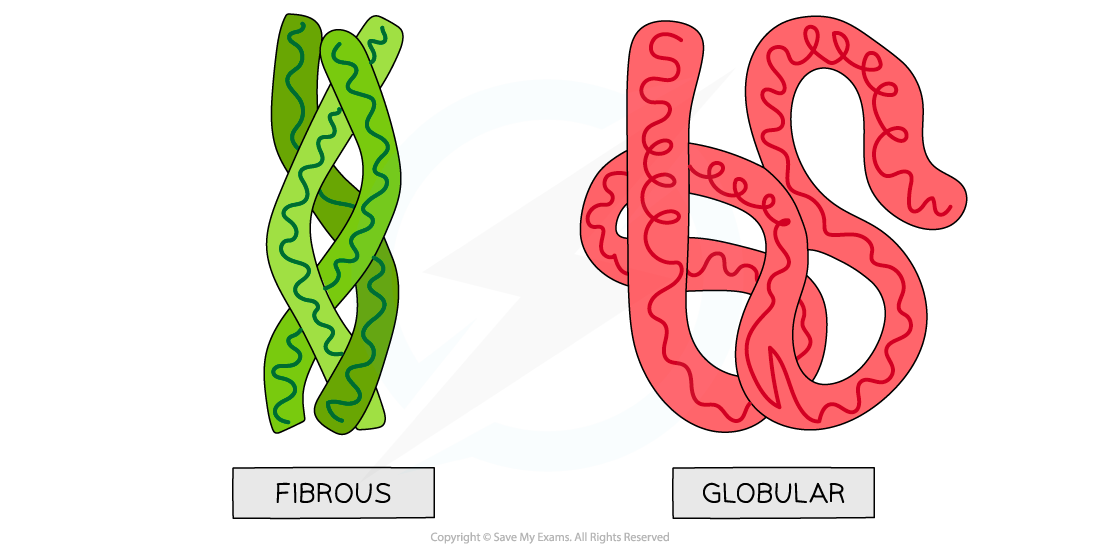
Globular and fibrous protein models illustrating the spherical shape of globular proteins and the long, stranded shape of fibrous proteins
Collagen
Collagen is the most common structural protein found in vertebrates
It provides structural support
In vertebrates it is the component of connective tissue which forms:
Tendons
Cartilage
Ligaments
Bones
Teeth
Skin
Walls of blood vessels
Cornea of the eye
Collagen is an insoluble fibrous protein
Structure of collagen
Collagen is formed from three polypeptide chains closely held together by hydrogen bonds to form a triple helix (known as tropocollagen)
Each polypeptide chain is a helix shape (but not α-helix as the chain is not as tightly wound) and contains about 1000 amino acids with glycine, proline and hydroxyproline being the most common
In the primary structure of collagen almost every third amino acid is glycine
This is the smallest amino acid with a R group that contains a single hydrogen atom
Glycine tends to be found on the inside of the polypeptide chains allowing the three chains to be arranged closely together forming a tight triple helix structure
Along with hydrogen bonds forming between the three chains there are also covalent bonds present
Covalent bonds also form cross-links between R groups of amino acids in interacting triple helices when they are arranged parallel to each other
The cross-links hold the collagen molecules together to form fibrils
The collagen molecules are positioned in the fibrils so that there are staggered ends (this gives the striated effect seen in electron micrographs)
When many fibrils are arranged together they form collagen fibres
Collagen fibres are positioned so that they are lined up with the forces they are withstanding
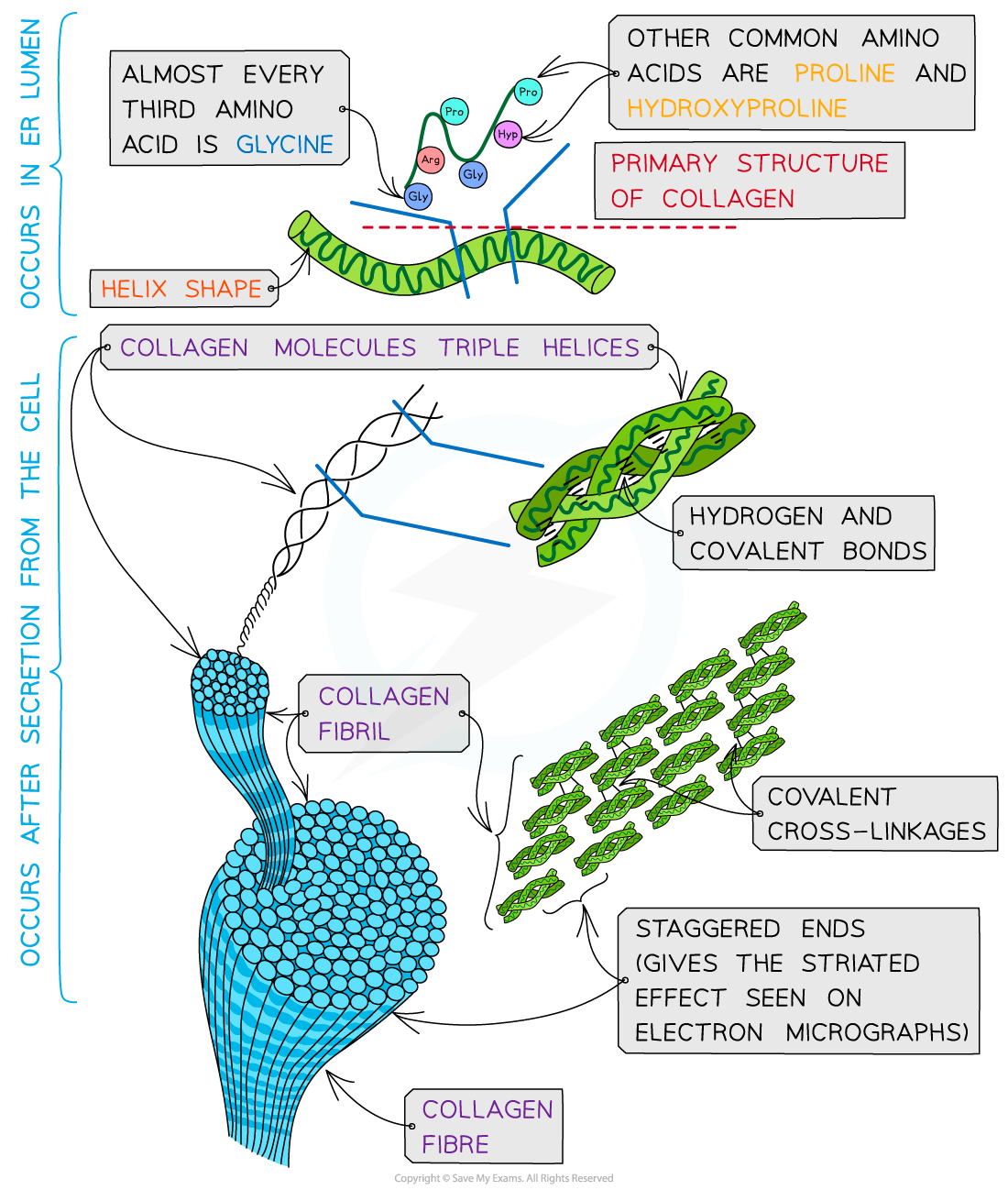
Collagen is a fibrous structural protein that is formed by triple helices. Collagen molecules arrange into collagen fibrils and finally into collagen fibres which have high tensile strength
Function of collagen
Collagen is a flexible structural protein forming connective tissues
The presence of the many hydrogen bonds within the triple helix structure of collagen results in great tensile strength. This enables collagen to be able to withstand large pulling forces without stretching or breaking
The staggered ends of the collagen molecules within the fibrils provide strength
Collagen is a stable protein due to the high proportion of proline and hydroxyproline amino acids present. These amino acids increase stability as their R groups repel each other
The length of collagen molecules means they take too long to dissolve in water (making it insoluble in water)
Comparison of Collagen & Haemoglobin Table
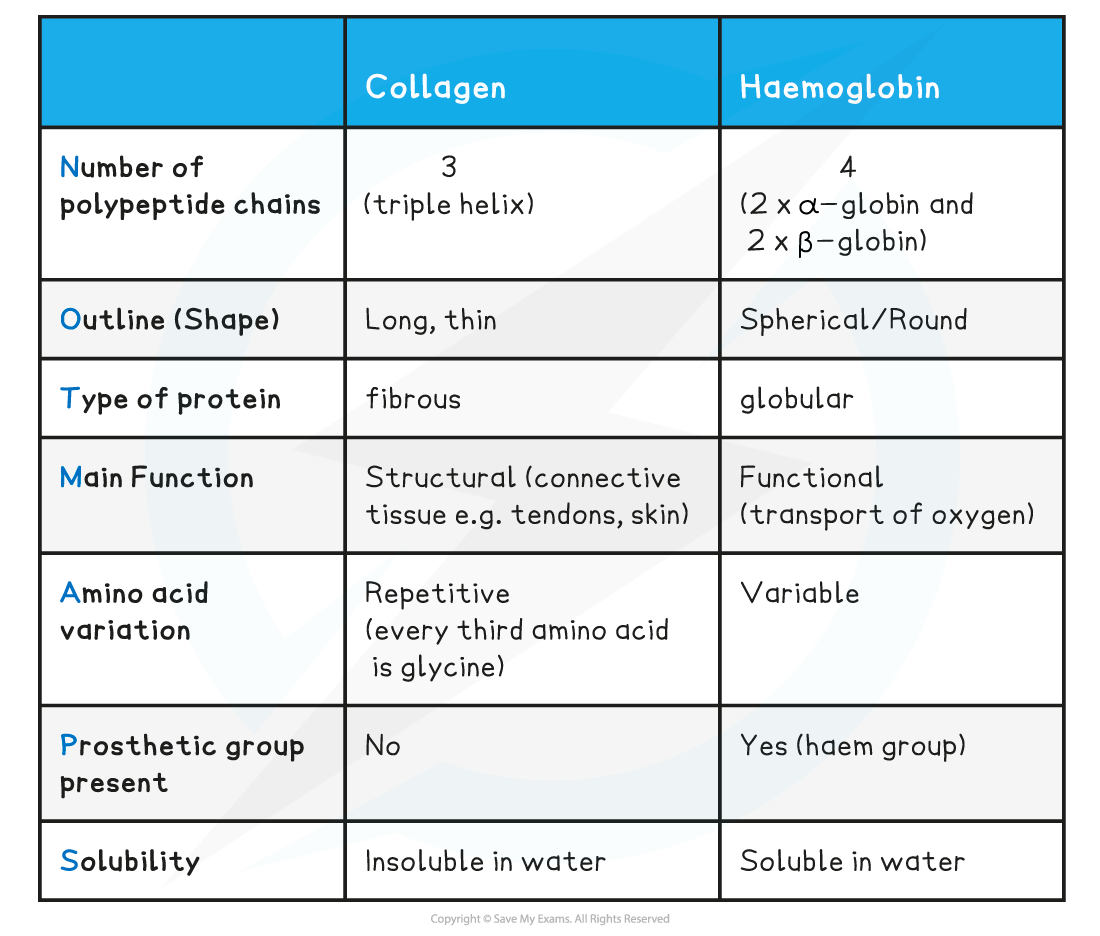
You've read 0 of your 5 free revision notes this week
Sign up now. It’s free!
Did this page help you?