Global Energy Balance Change (DP IB Geography): Revision Note
Variations in Solar Radiation
The Earth's energy budget (EEB) establishes Earth's climate
When the budget balances, temperatures on the Earth remain mostly constant
However, the incoming and outgoing energy don’t balance
The imbalance is partly caused by insolation, as it varies seasonally and with natural changes in the Earth's atmosphere
Changes in the make-up of the atmosphere alter the amount of energy absorbed and reflected
Changing factors such as greenhouse gases, water vapour etc., result in small, but significant energy imbalance on Earth
Other factors include:
Distance
Seasonal change
Latitude
Reflectiveness (albedo)
Factors affecting global insolation
Distance from the sun
Earth's orbit around the Sun is elliptical
Perihelion is when the Earth is closest to the Sun and insolation travels less distance
Aphelion is when the Earth is furthest away from the Sun - insolation has to travel further
Elliptical orbit of Earth
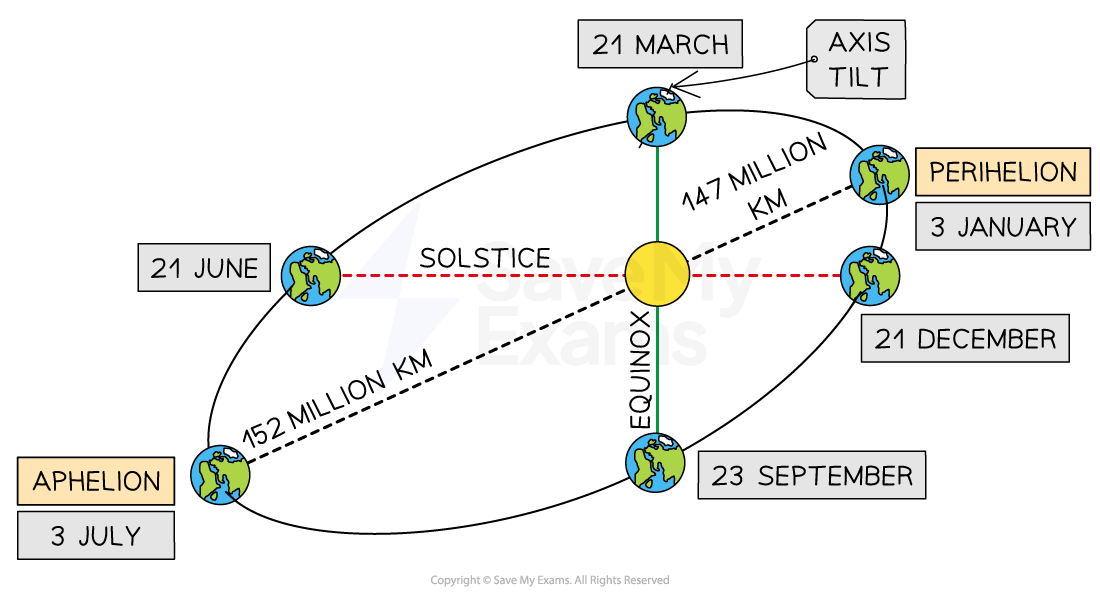
Latitudinal differences
Insolation has to pass through more atmosphere in the polar latitudes
Insolation is spread over a larger area in the polar regions
The Sun is overhead at the Equator and tropical latitudes receive more insolation
Uneven distribution of insolation
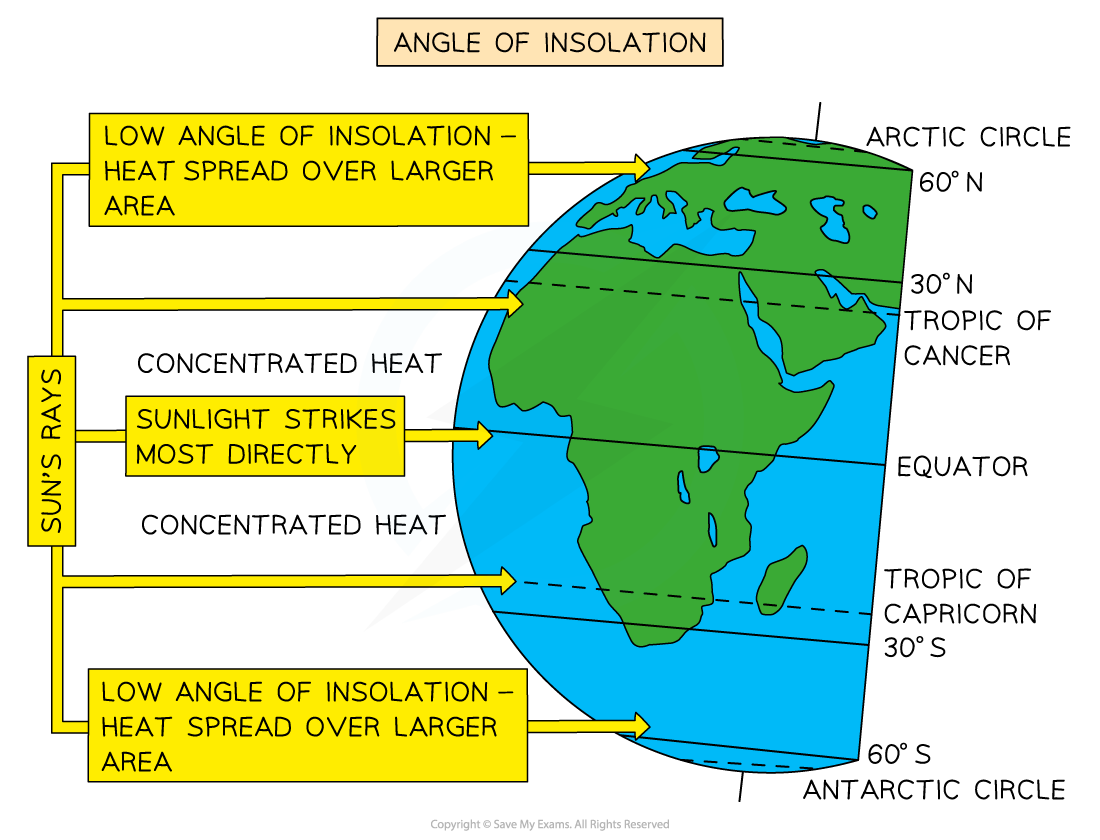
Seasonality and diurnal differences
The Earth is permanently tilted in the same direction on its axis
This tilt changes which hemisphere is facing the Sun as the Earth orbits throughout the year
This creates the seasons and daylight availability
Therefore, differences in the amounts of insolation gained or lost across the globe throughout the year
Seasonality affects global energy
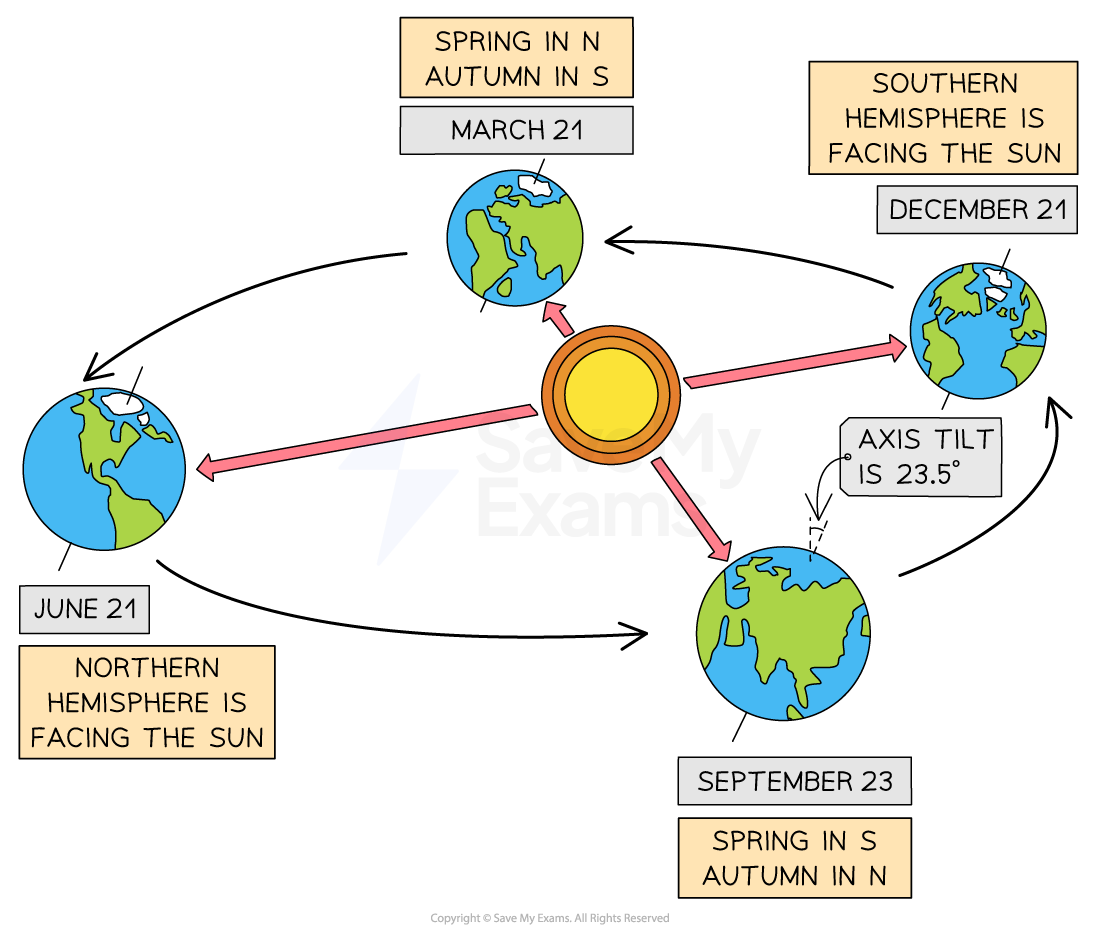
Milankovitch cycles
Milankovitch cycles describe the effects of changes in the Earth's movements on its climate over thousands of years
In the 1920s, Milankovitch suggested that variations in eccentricity, tilt, and wobble of the Earth's orbit resulted in cyclic changes in the amount of solar radiation reaching the Earth
Therefore, orbital changes influenced climatic patterns on Earth
Cycle | Time in Years (approx.) | Effect |
---|---|---|
Eccentricity (shape) | 100,000 | The Earth's orbit is currently elliptical making it closer to the Sun in January than in July. This results in the seasons being more extreme in the Southern Hemisphere than in the Northern Hemisphere. This shape will move to become more circular and this leads to cooler, even seasons, as the distance from the Sun will be more equal |
Obliquity (tilt) | 40,000 | If the Earth’s axis were vertical, there would be no seasons – the same part of the Earth’s surface would be facing the Sun throughout the year. The more angled the axis, the more extreme the seasons are (hotter summers and colder winters) |
Precession (wobble) | 26,000 | The axis also traces a circle in space and every 26,000 years the Earth wobbles on its axis and this changes which star we see as the North Star – currently it is Polaris, but 13,000 years ago, it would have been Vega |
Milankovitch cycles
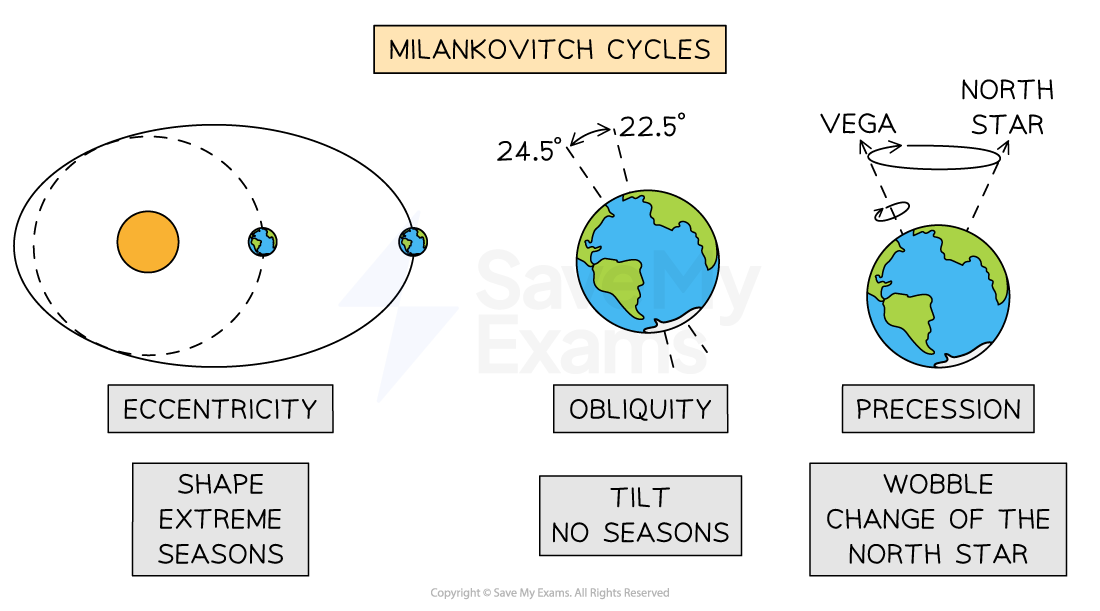
Sunspots and solar flares
Increased sunspot activity and solar flares are linked to higher average temperatures
Sunspots are areas of intense and complicated magnetic fields that emit solar plasma flares thousands of kilometres above the sun
The flare quickly rises to temperatures of 20 million °C
These bursts of high-energy radiation have the same energy as a few million volcanic eruptions on the Earth
Sunspots range from Earth-size 'pimples', to swollen scars halfway across the surface of the Sun
The Sun goes through 11-year cycles of solar activity
Ejection of solar plasma from the Sun
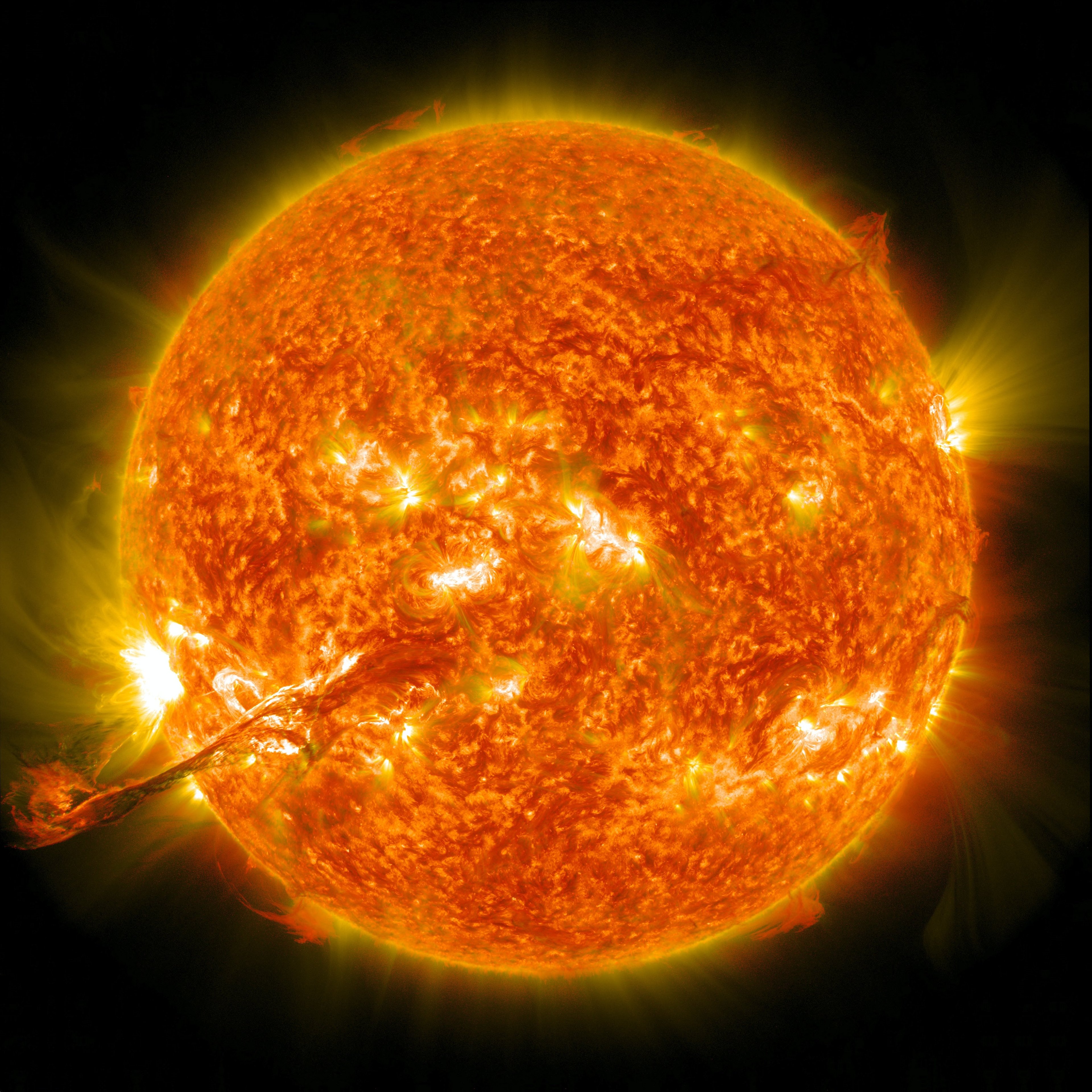
Photo by NASA on Unsplash
The more 'spots' on the Sun's surface, the higher the Sun's output
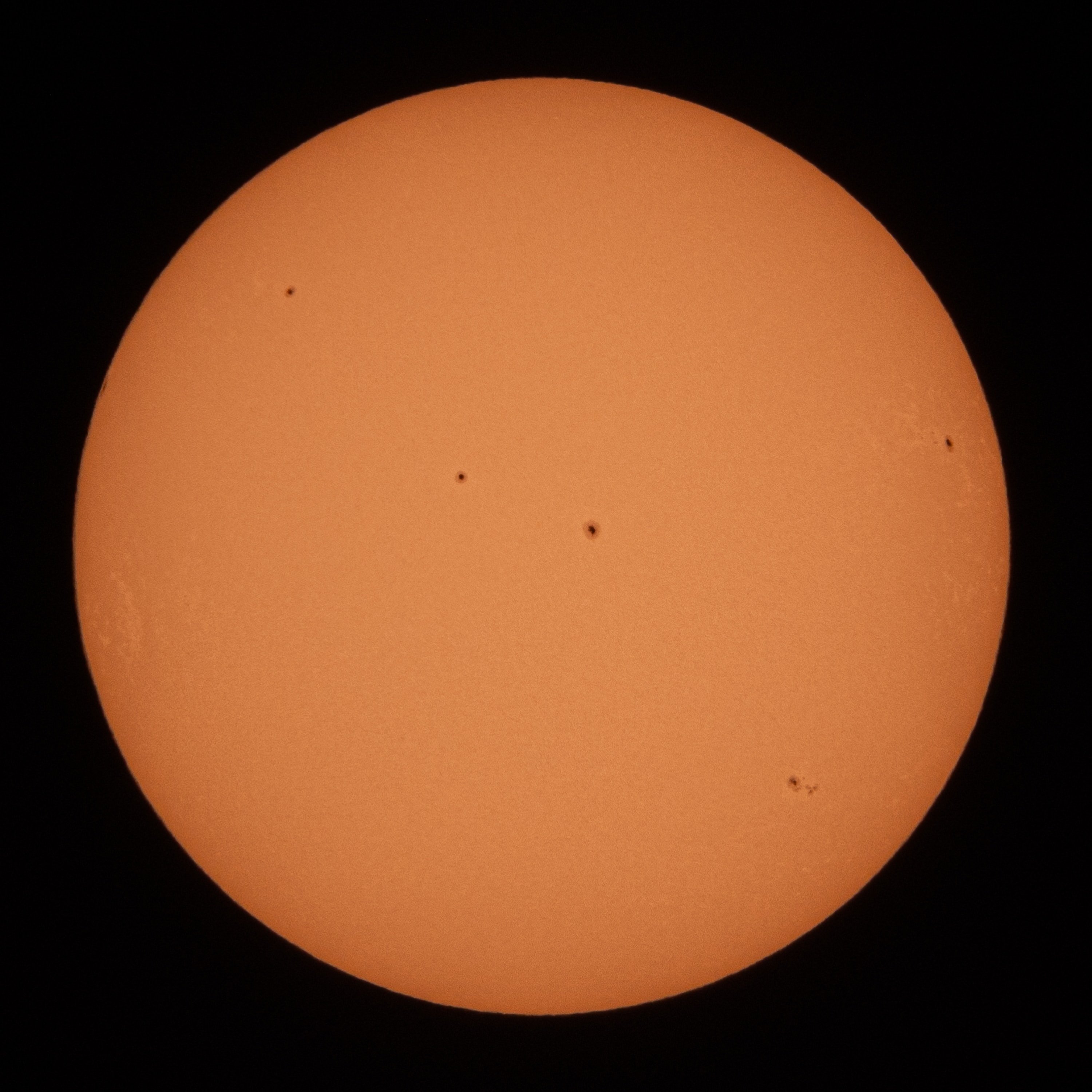
Photo by The Adaptive on Unsplash
Cloud cover
Clouds have higher albedos than the surface below, so more short-wave radiation is reflected back to space
Cloud cover at the equator reflects insolation – more is reflected having a net cooling effect
At the same time, clouds help contain the heat that would otherwise be emitted to space, through 'longwave warming,' which has a net warming effect
High, thin clouds, such as cirrus, allow insolation to pass through but absorb some long-wave radiation, warming the Earth’s surface
Deep convective clouds, especially cumulonimbus, neither heat nor cool overall
An overcast sky with complete cloud cover of low thick clouds – stratus and stratocumulus, can reflect 80% of insolation and cool the Earth’s surface
Global Dimming
Global dimming is caused by the increase of pollution in the atmosphere
Overall decline of 1-2% in insolation per decade since the 1950s
Between 1960 and 1990, the northern hemisphere saw a reduction of between 4% and 8% in insolation
Pollution controls in Europe and parts of North America have seen some recovery or global brightening
China and India have seen further, regional declines
Southern hemisphere is largely unaffected although increased development is having an impact
There are two timescales:
Short-term natural
Long-term anthropogenic
Short-term Natural Global Dimming
Cause | Impact |
---|---|
Volcanic eruptions | Large-scale eruptions block insolation and reduce temperatures The 1991 eruption of Mount Pinatubo, Philippines, reduced global temperatures by 1°C in 1992 |
Atmospheric dust and asteroids | Asteroids and meteors increase the amount of dust in the atmosphere, which decrease temperatures |
Wildfires | Wildfires have increased in size and intensity over the last decade. In 2020, wildfires burned more than a million acres in Oregon and more than 4 million acres in California. Although smoke eventually clears, it adds to global dimming because of fine matter and particles |
Long-term Anthropogenic Global Dimming
Cause | Impact |
---|---|
Aerosols | These are fine, solid particles or liquid droplets in the air and other gases. Most aerosols scatter light and some insolation back out to space, which exerts a cooling effect on the climate |
Particulate Matter | These include sulphur dioxide, ash, and soot, which are by-products of burning fossil fuels. Once in the atmosphere, they absorb and reflect insolation before it reaches the Earth's surface, causing dimming and cooling |
Water Droplets | Water droplets pick up particulates such as soot, ash, sulphur dioxide, etc. to form heavy, polluted 'brown clouds’. These clouds reflect light and energy back out to space, resulting in global dimming |
Vapour Trails (Contrails) | Contrail vapour from airplanes, flying high in the sky, reflect heat from the sun back out to space, causing global dimming |
Planetary Albedo
Planetary albedo is the amount of sunlight reflected from Earth's surface
Fresh snow and ice have the highest albedos, reflecting up to 95% of sunlight
Ocean surfaces absorb most sunlight, and so have low albedos
Hot bodies (sun) produce shortwave radiation, whereas, cold bodies (Earth) produce longwave radiation which is easily absorbed by GHGs and clouds
Positive & Negative Feedback
A feedback loop is a cycle within a system that either increases (positive) or decreases (negative) the effects on that system to achieve equilibrium
Positive feedback amplifies (enhances) a change and are destabilising
Negative feedback 'checks' or dampens change and are stabilising
Dynamic equilibrium
A system in a total state of balance is difficult to find, as nature is dynamic (ever changing)
Constant short-term adjustments are usually made through negative feedback to maintain balance
This process is referred to as 'dynamic equilibrium'.
Simple feedback system
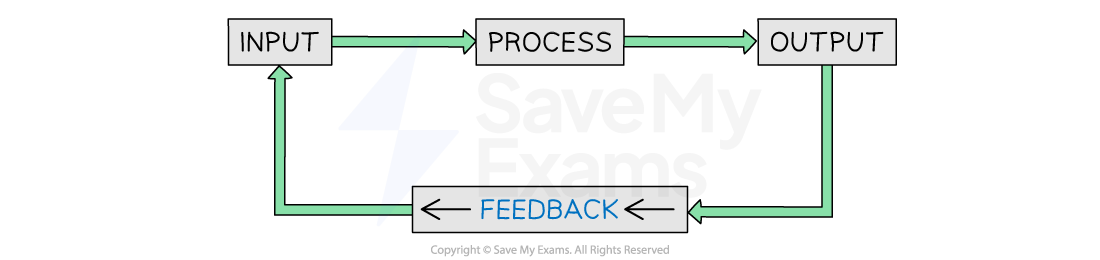
Example of a negative feedback loop
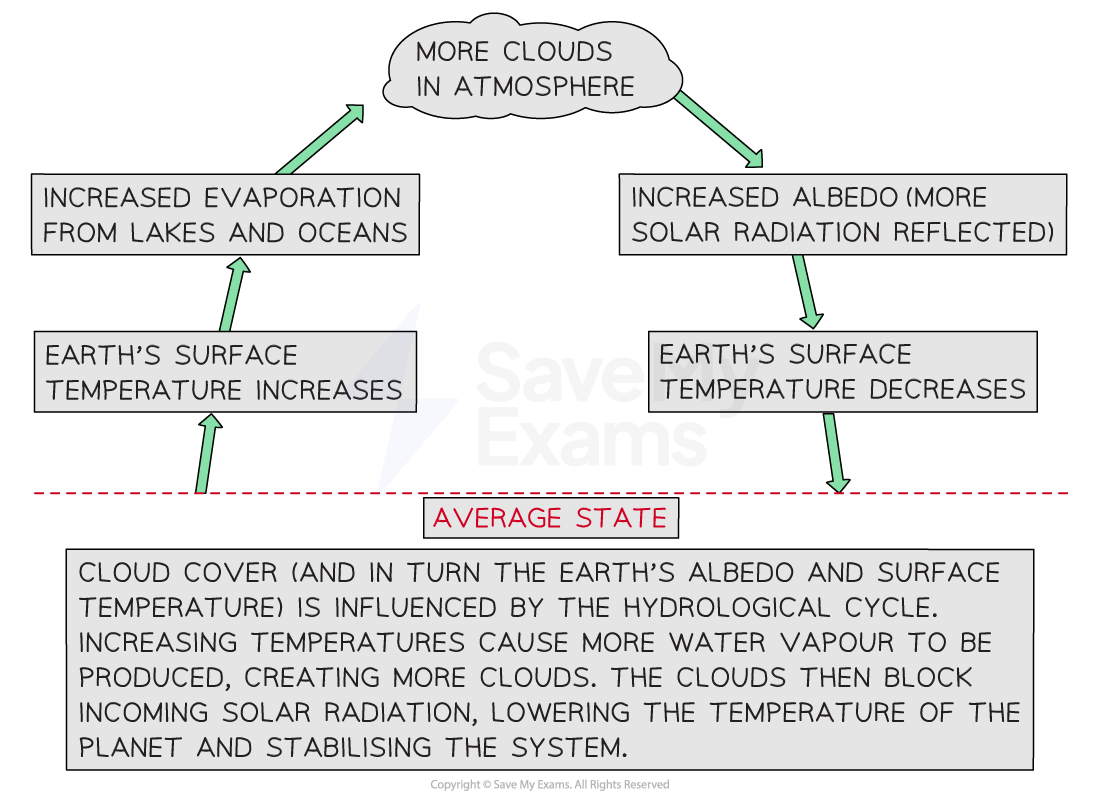
Many positive feedback loops contribute to global warming
Examples of positive feedback loops
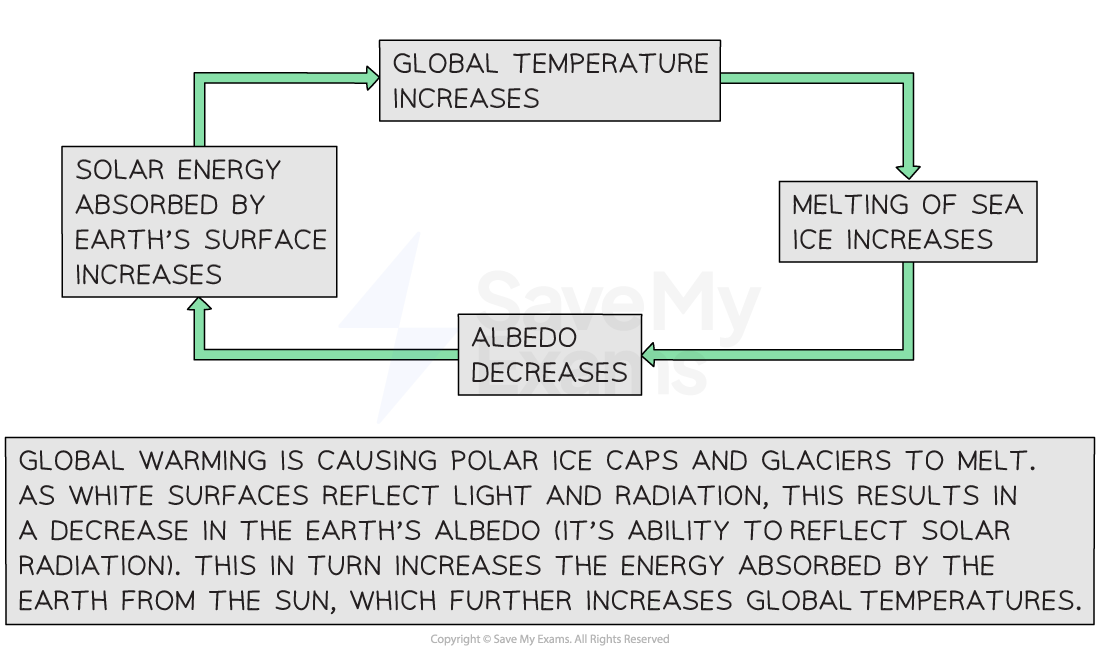
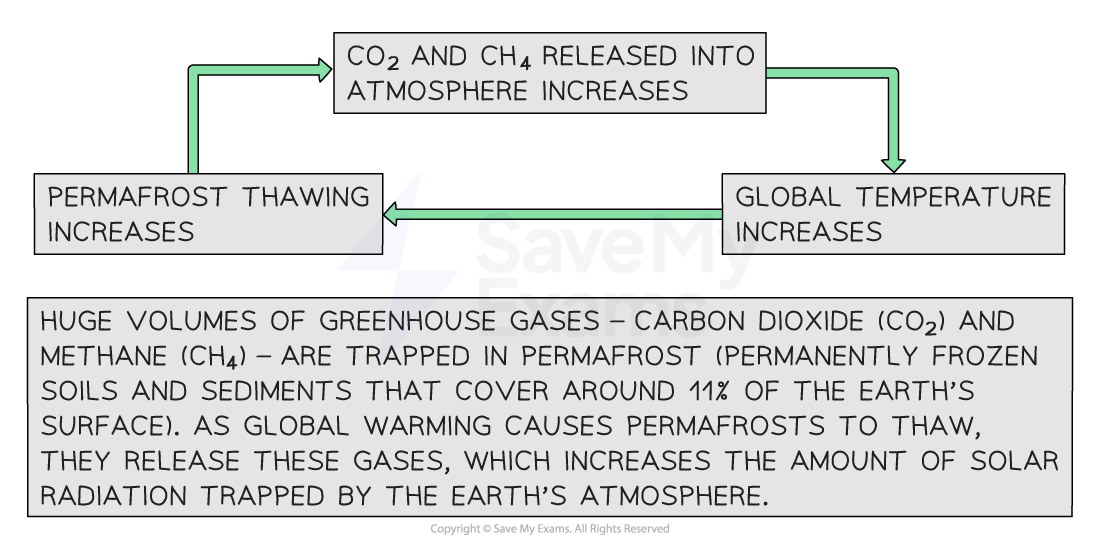
Examiner Tips and Tricks
Remember that a positive or negative feedback loop doesn't indicate whether the loop is good or bad.
In a system, a feedback loop is something that enhances or checks a process to bring the system back into balance.
You've read 0 of your 5 free revision notes this week
Sign up now. It’s free!
Did this page help you?