How Some Molecules Affect Gene Expression (College Board AP® Biology)
Study Guide
Written by: Phil
Reviewed by: Lára Marie McIvor
Regulatory DNA Sequences
The nucleus of every cell in the human body contains the same genes
However not every gene is expressed in every cell
Different genes are expressed at different times in different cells
This is called differential gene expression and is fundamental in allowing cells to become specialized for particular functions
Several mechanisms exist within cells to make sure the correct genes are expressed in the correct cell at the right time
They involve regulatory genes and regulatory sequences
Structural & regulatory genes
A structural gene codes for a protein that has a function within a cell
For example, the F8 gene codes for the protein Factor VIII involved in blood clotting
A regulatory gene codes for a protein that helps to control the expression of another gene
A regulatory sequence is a stretch of DNA where regulatory proteins can bind to control transcription
Structural and regulatory genes that work together are usually found close together
Regulatory sequences are located upstream of the gene to be transcribed
An Example of Differential Gene Expression
Inducible & repressible enzymes
Some genes code for proteins that form enzymes
Some enzymes are required all the time and some are required only at specific times
The expression of enzyme producing genes can be controlled
Inducible enzymes are only synthesized when their substrate is present
The presence of the substrate induces the synthesis of of the enzyme by causing the transcription of the gene for the enzyme to start
Repressible enzymes are synthesized as normal until a repressor protein binds to an operator
The presence of the repressor protein represses the synthesis of the enzyme by causing the transcription of the gene for the enzyme to stop
Controlling when enzymes are synthesized can be beneficial for cells as it stops materials and cellular energy being wasted
For example, using materials and energy to synthesize an enzyme when its substrate is not present and it can’t carry out its function would be highly wasteful
Epigenetics
An organism’s internal or external environment can influence gene expression patterns
The levels of regulatory proteins or transcription factors can be affected in response to environmental stimuli such as light and chemicals, including drugs and hormones
For example, enzymes are activated in response to ultraviolet radiation and increase the expression of melanin-producing genes (and therefore production of melanin), leading to skin pigmentation
Epigenetics is the control of gene expression by factors other than an individual’s DNA sequence
Epigenetics involves the switching on and switching off of genes, but without changing the actual genetic code
In eukaryotic cells, nuclear DNA is wrapped around proteins called histones to form chromatin
Chromatin can be chemically modified in different ways to alter gene expression, including:
Methylation of DNA (chemical addition of -CH3 groups)
Histone modification via acetylation of amino acid tails
Such modifications are called epigenetic tags and collectively, all the epigenetic tags in an organism is called the epigenome
The epigenome can undergo changes due to environmental factors
Smoking, stress, exercise and diet can cause epigenetic changes
Internal signalling from the body's own cells can also cause modifications to occur
Epigenetic modification is independent (ie. DNA methylation or histone modification in one area is not linked to modification in another)
The chemical modification of histones and DNA controls how tightly the DNA is wound around them as the intermolecular bonding between the histones and DNA changes
If the DNA is wound more tightly in a certain area, the genes on this section of DNA are switched off as the gene and promoter regions are more hidden from transcription factors and RNA polymerase
The modification of histones is reversible and therefore can be different in different cell types and can vary with age
Structure of Chromatin Diagram
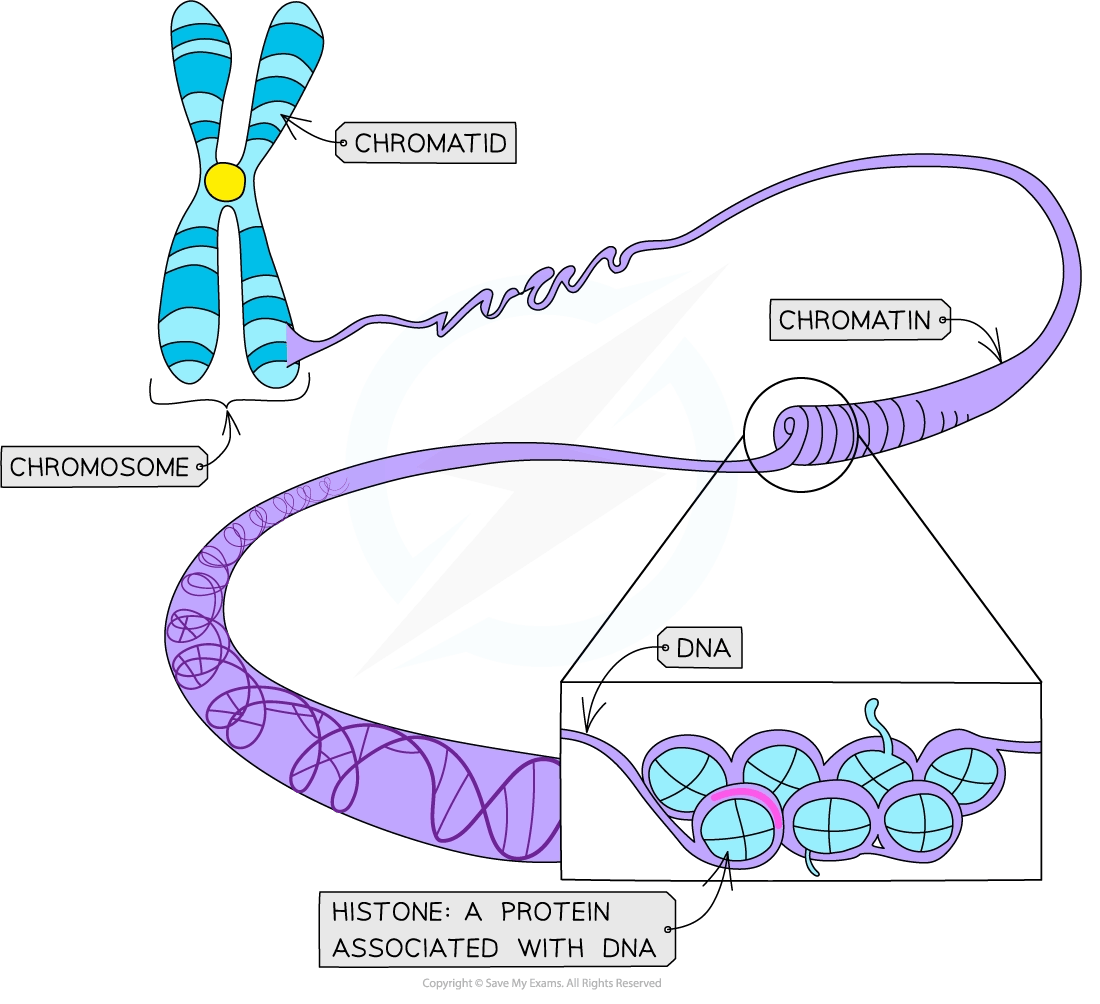
DNA is coiled around histone proteins to make chromatin
DNA methylation causes the inactivation of genes
One method of epigenetic control involves the methylation of DNA
Methyl groups (-CH3) can be directly added to DNA to change the activity of a gene
DNA methylation commonly involves the direct addition of a methyl group to cytosine bases which can influence gene expression
Methylation of DNA suppresses the transcription of the affected gene by inhibiting the binding of transcription factors and enzymes needed for transcription (e.g. RNA polymerase)
Cells use this mechanism to lock genes in the ‘off’ position
The gene is said to be repressed or inactivated
DNA methylation can be affected by many environmental, lifestyle or age-related factors
Acetylation of histones affects gene expression
Acetyl groups (-COCH3) can be added to lysine amino acids within histone proteins
Lysine has a positively charged R group, this forms ionic bonds with the negatively charged phosphate backbone of DNA
This helps DNA to coil tightly around the histone protein core
Adding acetyl groups (acetylation) to lysine removes the positive ion and therefore removes a bond between the histone protein and the DNA, this causes the DNA to be less tightly wrapped
When the DNA is less tightly wrapped, RNA polymerase and transcription factors can bind more easily and therefore gene expression can occur
The gene is said to be activated
Removal of acetyl groups (deacetylation) returns lysine to its positively charged state which has a stronger attraction to the DNA molecule and therefore inhibits transcription and once again stops the gene from being expressed
Acetylation of Histones Diagram
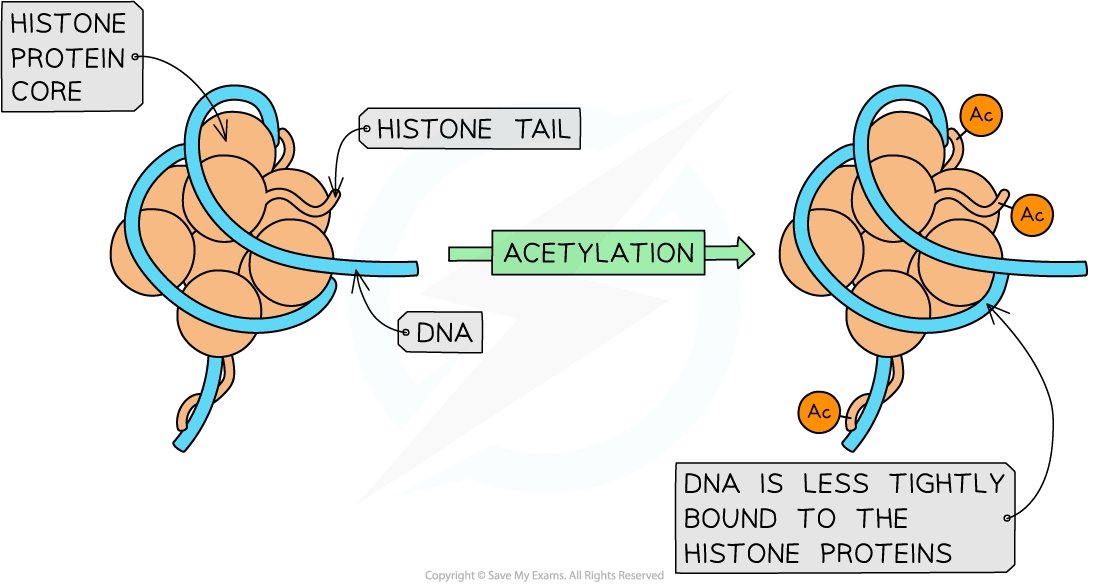
Acetylation of histones causes chromatin to become less condensed, allowing genes to be transcribed
Epigenetic changes can be passed on following cell division
Like the genome, the epigenome is heritable (i.e. when a cell divides and replicates, epigenetic changes affecting the expression of genes in the DNA of that cell may be passed on to daughter cells)
For example, during gamete production, DNA in the parent cell usually undergoes demethylation (the methyl groups are removed) but often methyl groups are not removed and therefore are present in the DNA on the sperm or egg cells
One potential explanation for why this occurs is that if an epigenetic change occurs in response to an environmental factor, it may be beneficial for this epigenetic change to also occur in daughter cells (or gametes, for example) so that they are also better adapted for the environmental factor (in the same way the parent cell was)
Mounting evidence demonstrates that modifications to the epigenome in one generation can be passed on to the next generation at the cellular or whole organism level
Examiner Tips and Tricks
Epigenetics can be distinguished from mutations, both of which lead to changes in the expression of genes - whilst mutations affect the genetic code itself, by altering nucleotide sequences, epigenetic changes only affect the way the code is read.
Examples of Regulatory Sequences in Organisms
The lac operon
Both prokaryotes and eukaryotes have groups of genes that are regulated together
In prokaryotes, groups of genes called operons are transcribed in a single mRNA molecule
The lac operon is an example of an inducible system
Regulatory genes control structural genes and their levels of protein production
Regulatory genes sometimes have control over several structural genes at once
Structural genes in prokaryotes can form an operon: a group or a cluster of genes that are controlled by the same promoter
The lac operon found in some bacteria is one of the most well known of these
The lac operon controls the production of the enzyme lactase (also called β-galactosidase) and two other structural proteins
Lactase breaks down the substrate lactose so that it can be used as an energy source in the bacterial cell
It is an inducible enzyme that is only synthesized when lactose is present
This helps prevent the bacteria from wasting energy and materials
Structure of the lac operon
The components of the lac operon are found in the following order:
Promoter for structural genes
Operator
Structural gene lacZ that codes for lactase
Structural gene lacY that codes for permease (allows lactose into the cell)
Structural gene lacA that codes for transacetylase
Located to the left (upstream) of the lac operon on the bacterium's DNA there is also the:
Promoter for regulatory gene
Regulatory gene lacI that codes for the lac repressor protein
The lac repressor protein has two binding sites that allow it to bind to the operator in the lac operon and also to lactose (the effector molecule)
When it binds to the operator it prevents the transcription of the structural genes as RNA polymerase cannot attach to the promoter
When it binds to lactose the shape of the repressor protein distorts and it can no longer bind to the operator
The Structure of the lac Operon
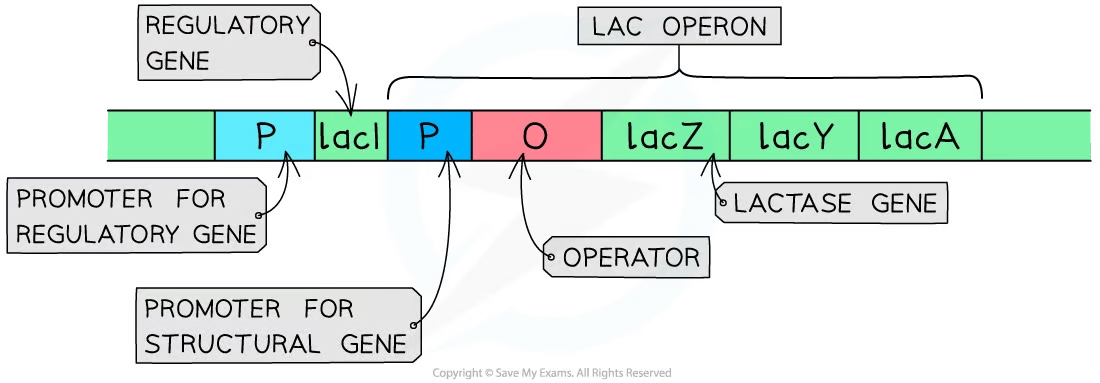
The components of the lac operon along with the upstream regulatory gene and its associated promoter
When lactose is absent
The following processes take place when lactose is absent in the medium that the bacterium is growing in:
The regulatory gene is transcribed and translated to produce lac repressor protein
The lac repressor protein binds to the operator region upstream of lacZ
Due to the presence of the repressor protein RNA polymerase is unable to bind to the promoter region
Transcription of the structural genes does not take place
No lactase enzyme is synthesized
Lac Operon in the Absence of Lactose Diagram
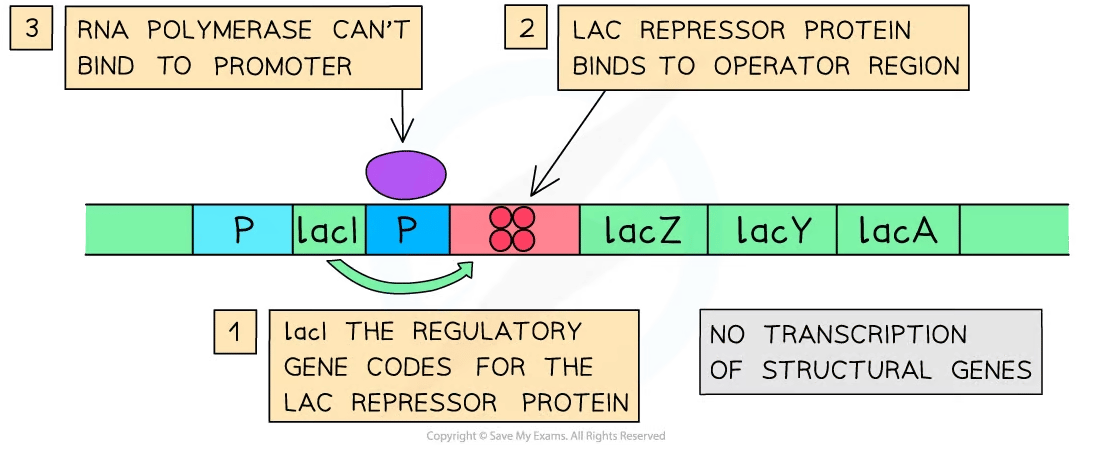
The repressor protein binding to the operator region of the lac operon and preventing transcription of the structural gene
When lactose is present
The following processes take place when lactose is present in the medium that the bacterium is growing in:
There is an uptake of lactose by the bacterium
The lactose binds to the second binding site on the repressor protein, distorting its shape so that it cannot bind to the operator site
RNA polymerase is then able to bind to the promoter region and transcription takes place
The mRNA from all three structural genes is translated
Enzyme lactase is produced and lactose can be broken down and used for energy by the bacterium
Lac Operon in the Presence of Lactose Diagram
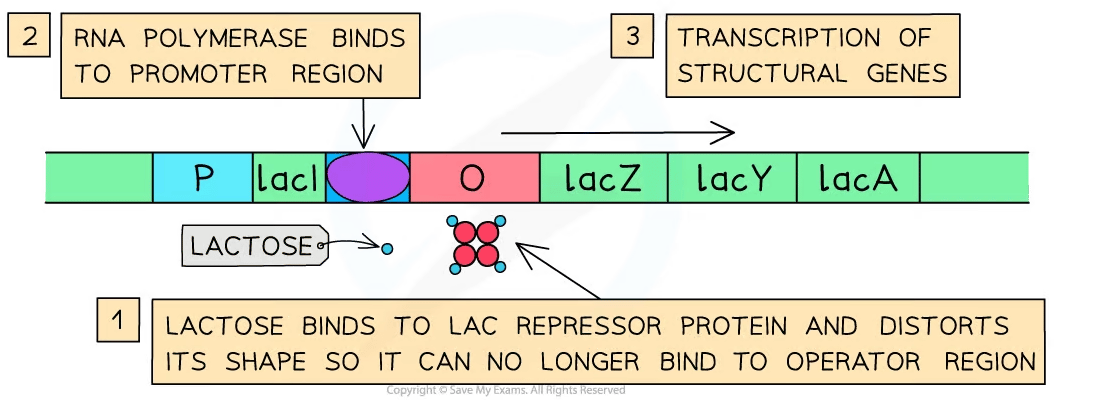
Lactose binding to the repressor protein which frees up the operator region of the lac operon so RNA polymerase can bind and begin transcription of the structural genes
Regulation of Gene Expression in Eukaryotes
In eukaryotes, groups of genes may be influenced by the same transcription factors to regulate expression in a coordinated manner
Transcription factors bind to regulatory sequences in the DNA molecule and in doing so, control whether a gene is expressed or not
For example, one single transcription factor could control the expression of a group of genes that are distinct yet all involved in the coordinated response to a certain external stimulus, like a feeding response to a change in nutrient availability
Examiner Tips and Tricks
The example above explains how the genetic control of an inducible enzyme works. You could get some questions on the genetic control of repressible enzymes.
In this mechanism an effector molecule also binds to a repressor protein produced by a regulatory gene. However this binding actually helps the repressor bind to the operator region and prevent transcription of the structural genes. So it's the opposite of the lac operon: when there is less of the effector molecule, the repressor protein cannot bind to the operator region and transcription of the structural genes goes ahead, meaning the enzyme is produced.
Last updated:
You've read 0 of your 5 free study guides this week
Sign up now. It’s free!
Did this page help you?