Mendel's Laws (College Board AP® Biology)
Study Guide
Written by: Phil
Reviewed by: Lára Marie McIvor
Mendel's Laws
Gregor Mendel was an Austrian monk
In the mid-19th century, Mendel carried out breeding experiments on large numbers of pea plants whilst looking after the monastery gardens where he lived
He studied how characteristics were passed on between generations of plants
Due to his extensive work on the understanding of inheritance, he is sometimes called the Father of Genetics
Mendel artificially pollinated the plants to allow sexual reproduction
He did this by carefully transferring pollen (the male gamete) from the anthers of one pea plant to the ovary (female reproductive parts) of another
This technique eliminated any uncertainty from his data since he knew which pollen had fertilized each of the plants
He collected the pea seeds from these plants and grew them in favorable conditions to find out their characteristics
He also crossbred offspring peas in order to find out which, if any characteristics would appear in future generations
Mendel investigated the height of pea plants, the colors of their flowers and the smoothness of their seed coats
Mendel's Breeding Experiments of Pea Plants Diagram
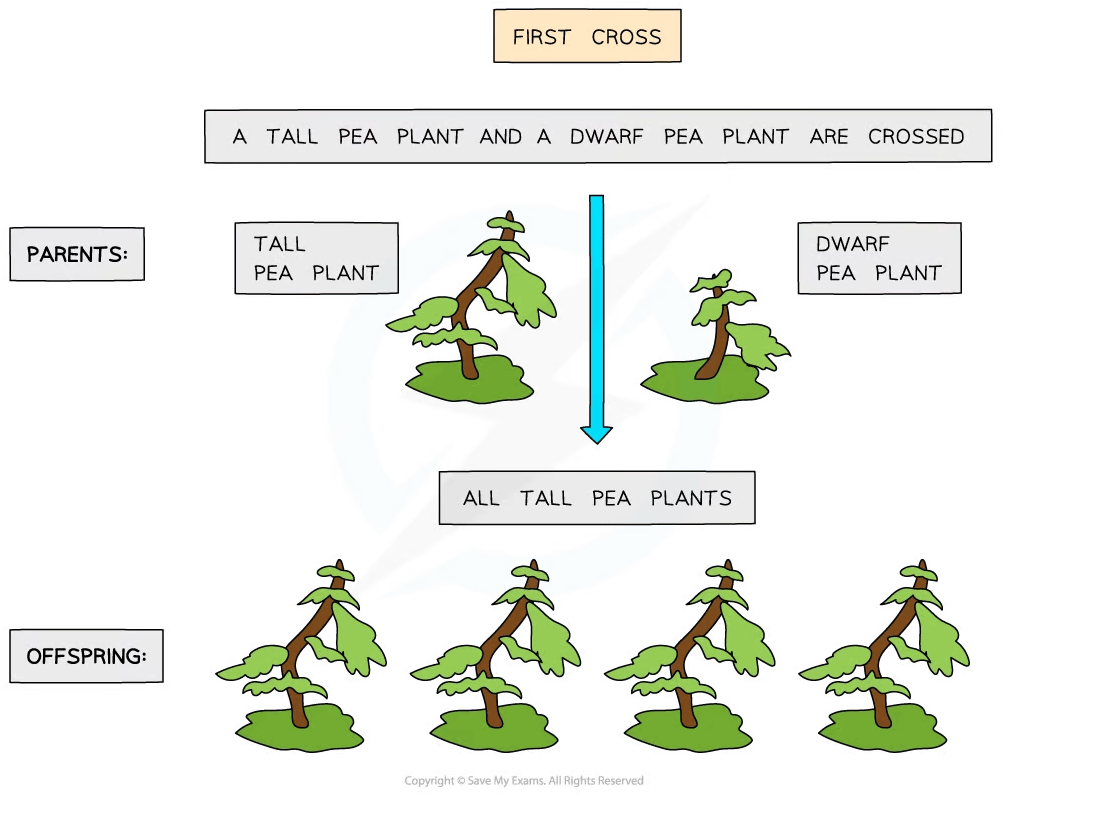
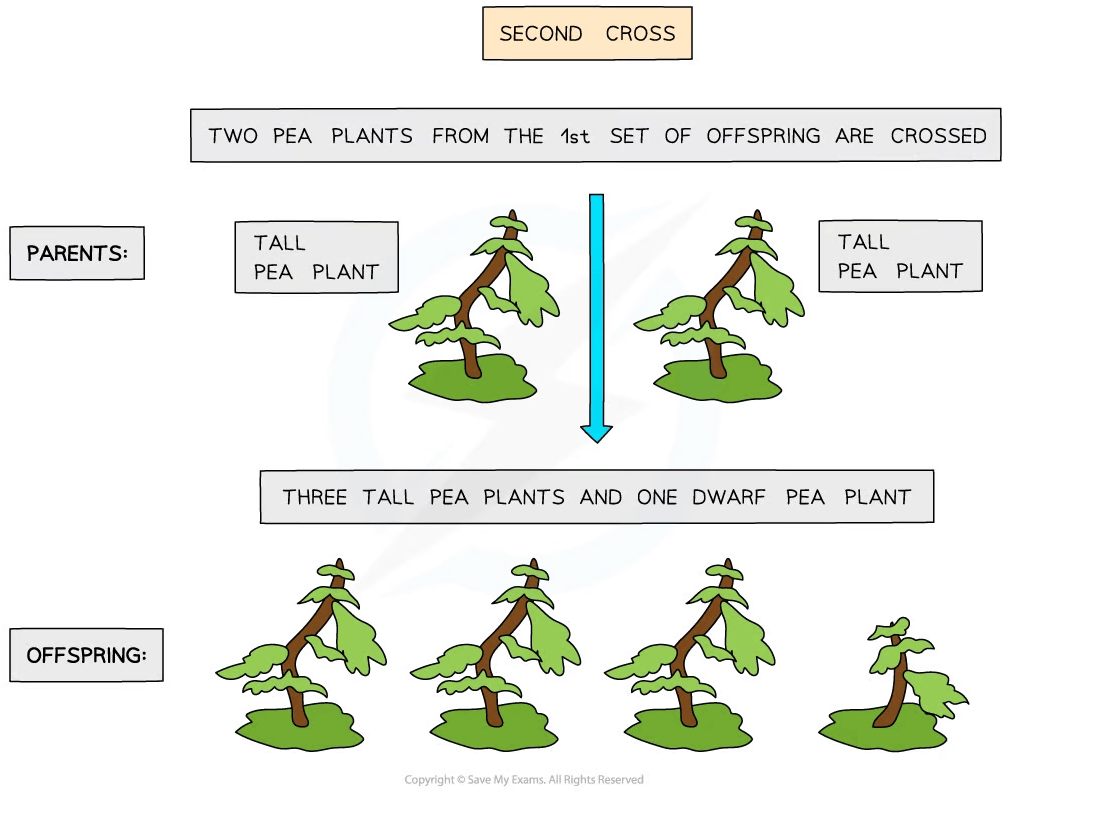
In this cross, Mendel investigated the inheritance of height in pea plants
Mendel found that characteristics were inherited in a predictable pattern
All pea plants in the first generation had the same characteristic as one of the parental plants (e.g. they were all tall)
The offspring plants in the second generation had characteristics of both parent plants in a 3:1 ratio
Without knowing it, Mendel had discovered genes, he referred to them as 'units of inheritance'
He also discovered that some genes are dominant and some genes are recessive
Different forms of the same gene are called alleles
These observations formed the basis of Gregor Mendel's laws of inheritance
Mendel's First Law - The Law of Segregation
Each trait is determined by two alleles that are randomly separated during gamete formation
Gametes contain one allele of each gene
This means there are two possible allele outcomes for each gene in each gamete
During fertilization, two gametes fuse to restore the diploid number of alleles - two alleles for each gene
Combinations of alleles in offspring are random due to random segregation and random fertilization
Mendel's Second Law - The Law of Independent Assortment
Genes located on different chromosomes are assorted independently during gamete formation
There are 23 pairs of chromosomes and each homologous pair contains a different set of genes
The inheritance of traits on one chromosome does not influence which traits are inherited on another chromosome
The alleles of a gene are segregated randomly into different gametes and the assortment of alleles of one gene does not affect the assortment of alleles for another gene
During fertilization, two gametes fuse to restore the diploid number of alleles - two alleles for each gene
Combinations of alleles from different genes in offspring are random due to random assortment and random fertilization
Examiner Tips and Tricks
Mendel's Laws of inheritance applied only to unlinked genes on different chromosomes e.g. one gene found on chromosome 1 and another found on chromosome 2.
Genes located on the same autosome are called 'autosomal linked' genes
Genes located on the sex chromosomes are called 'sex-linked' chromosomes
Monohybrid Crosses
Monohybrid Inheritance
The rules of probability can be applied to predict the inheritance of single gene, or monohybrid, traits
In a monohybrid cross, we consider that the gene has two alleles
Where one allele is dominant and the other is recessive
Sometimes the alleles are codominant
To predict the outcome of a monohybrid cross, we start with purebreeding parents (homozygous), each displaying a different phenotype
Homozygous dominant and homozygous recessive
This generation is known as the parental generation, denoted as the P generation
We can use a Punnett grid to predict the probability of a certain offspring, of the P generation, displaying a certain genotype or phenotype
Steps in Constructing a Punnett Grid
Write down the parental phenotypes and genotypes
Write down all the possible gamete genotypes that each parent could produce for sexual reproduction
A useful convention is to write the gamete genotypes inside a circle to denote them as gametes (haploid cells)
Place each parental genotype against one axis of a Punnett grid (2 x 2 table)
In the boxes of the Punnett grid, combine the gametes into the possible genotypes of the offspring
This gives the offspring of the F1 generation (1st filial generation)
List the phenotype and genotype ratios for the offspring
Worked Example
Sweet peas grow pods that are either green or yellow. The allele for green, G, is dominant to the allele for yellow, g. Construct a Punnett grid to predict the outcome when crossing green and yellow pure-bred plants to show the F1 generation offspring. Using plants from the F1 generation, construct a second Punnett grid to show the outcomes of the F2 generation.
Step 1: Write down the parental phenotype and genotypes
Green coloured pods Yellow coloured pods
GG gg
Step 2: Write down all the possible gamete genotypes that each parent could produce
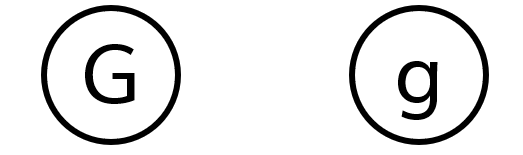
Step 3: Place each parental genotype against one axis of a Punnett grid (2 x 2 table)
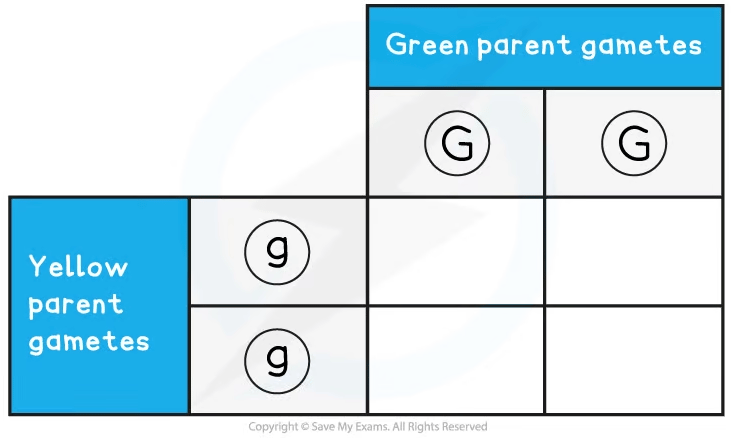
Step 4: Combine the gametes in each box of the Punnett grid
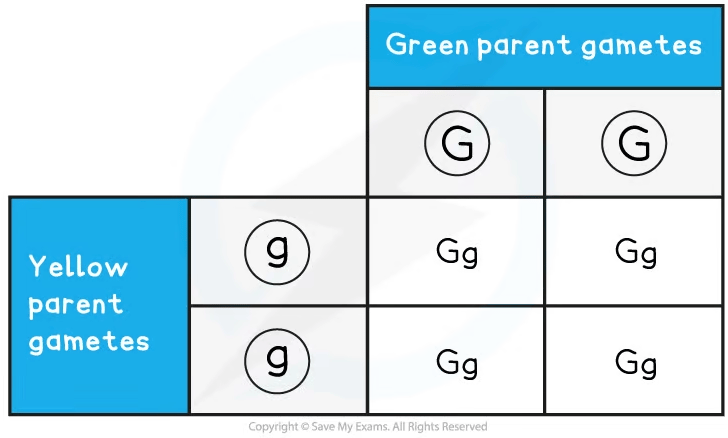
Genotypes of the F1 cross between homozygous green (GG) and homozygous yellow (gg) pea plants.
All offspring (100%) have the genotype Gg and the phenotype is green.
Step 5: Take two heterozygous offspring from the F1 generation and cross them
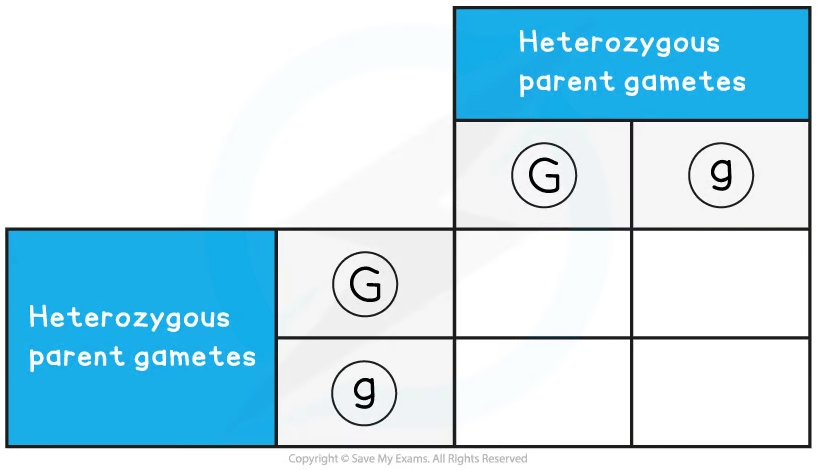
Step 6: Combine the gametes in each box of the Punnett grid
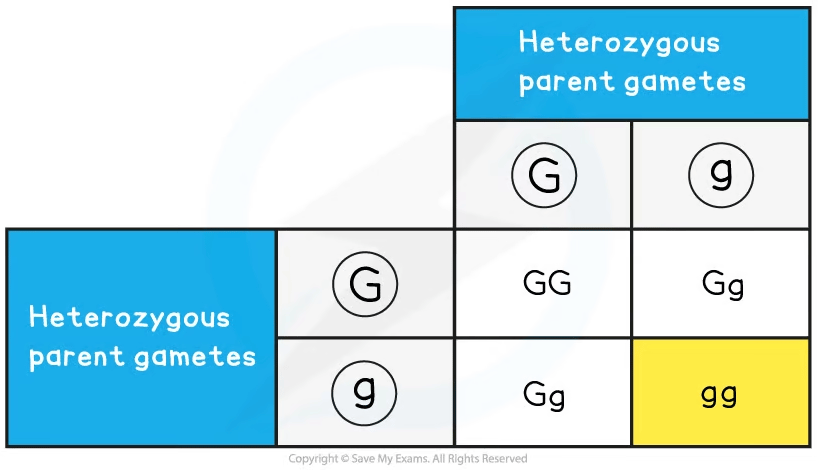
Punnett grid showing the results of the F2 generation
Phenotype ratio is 3:1 green: yellow, Genotype ratio is 1 GG: 2 Gg: 1 gg
Dihybrid Crosses
Monohybrid crosses look at how the alleles of one gene transfer across generations
Dihybrid crosses look at how the alleles of two genes transfer across generations
i.e. dihybrid crosses can be used to show the inheritance of two completely different characteristics in an individual, for example unlinked genes
The genetic diagrams for both types of cross are very similar
For dihybrid crosses, there are several more genotypes and phenotypes involved
When writing out the different genotypes, write the two alleles for one gene, followed immediately by the two alleles for the other gene
Do not mix up the alleles from the different genes
For example, if there was a gene with alleles Y and y and another gene with alleles G and g an example genotype for an individual would be YyGg
Alleles are usually shown side by side in dihybrid crosses e.g. TtBb
Worked Example
Worked example 1: Dihybrid genetic diagram
Horses have a single gene for coat colour that has two alleles:
B, a dominant allele produces a black coat
b, a recessive allele produces a chestnut coat
Horses also have a single gene for eye colour
E, a dominant allele produces brown eyes
e, a recessive allele produces blue eyes
Each of these genes (consisting of a pair of alleles) are inherited independently of one another because the two genes are located on different non-homologous chromosomes
Such characteristics are said to be unlinked
In this example, a horse that is heterozygous for both genes has been crossed with a horse that is homozygous for one gene and heterozygous for the other
Parental phenotypes: | black coat, brown eyes | x | chestnut coat, brown eyes |
Parental genotypes: | BbEe | x | bbEe |
Parental gametes: | BE or Be or bE or be | x | bE or be |
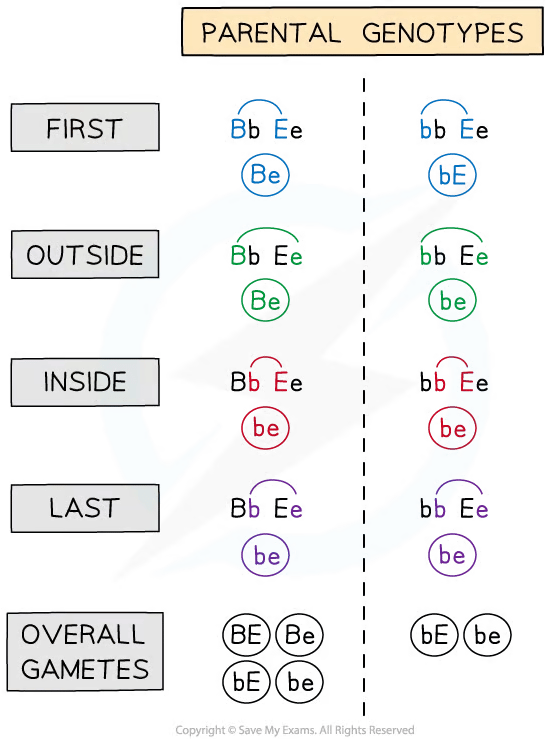
Determining the Alleles Carried by Gametes Based on the Parental Genotypes Using the FOIL (First, Outside, Inside, Last) Method
Dihybrid Cross Punnett Square Table
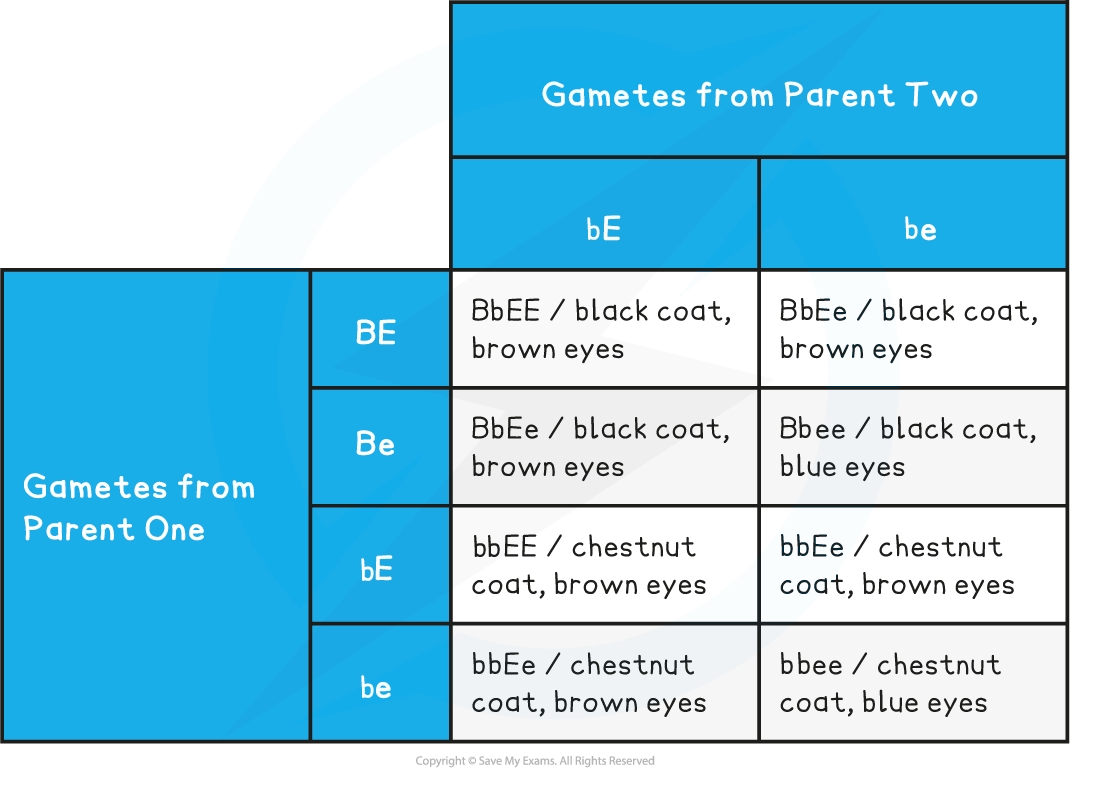
Predicted ratio of phenotypes in offspring
3 black coat, brown eyes :
3 chestnut coat, brown eyes :
1 black coat, blue eyes :
1 chestnut coat, blue eyes
Predicted ratio of genotypes in offspring = 3 BbEE : 3 bbEE : 1 Bbee : 1 bbee
Laws of Probability
Rules of probability (as in the worked example above) can be applied to analyze the inheritance of single gene characteristics from parent to offspring; this is called monohybrid inheritance
If events A and B are mutually exclusive, then:
P(A or B) = P(A) + P(B)
If events A and B are independent, then:
P(A and B) = P(A) × P(B)
It is important to remember that the rules of probability will only apply accurately to unlinked genes
However the data yielded by crossing experiments and formation pedigree diagrams (with the genotypes and phenotypes of parents and offspring) can lead to conclusions that support other types of inheritance:
Dihybrid inheritance
Linked inheritance (genes on the same chromosome in pairs 1 - 22 in humans)
Sex-linked inheritance
Genetic Pedigree Diagram
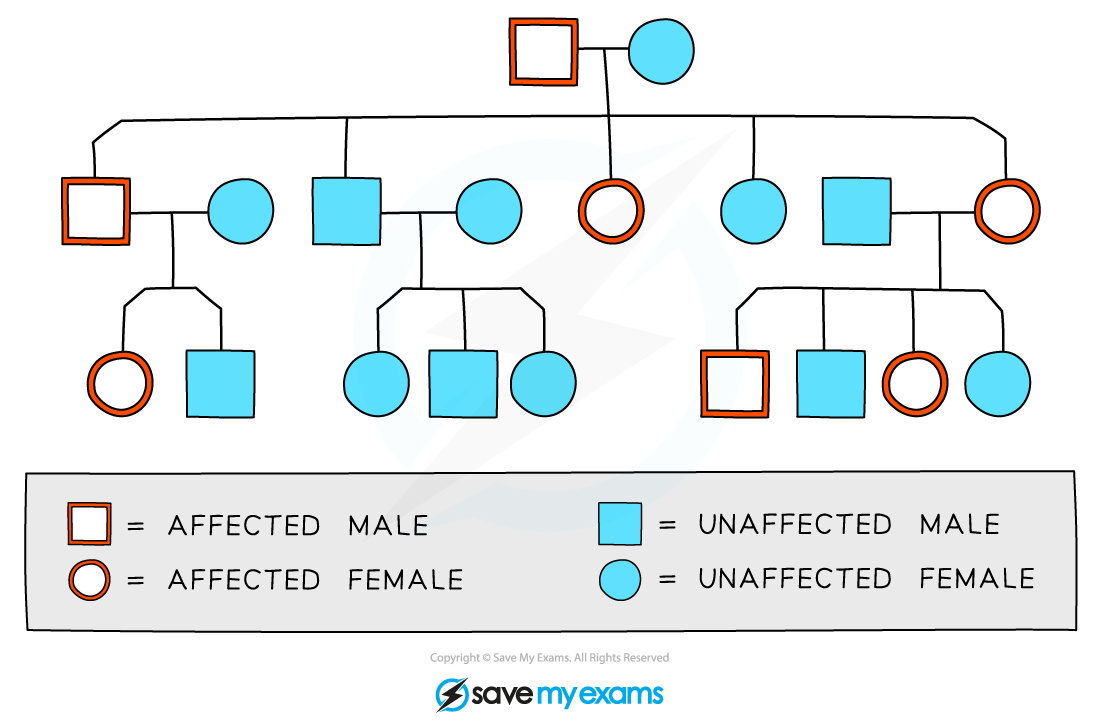
The data contained in a pedigree diagram allows patterns of inheritance to be mapped out and traced to predict the inheritance of genes even if they are linked-genes
Last updated:
You've read 0 of your 5 free study guides this week
Sign up now. It’s free!
Did this page help you?