Threshold Frequency & Work Function (AQA A Level Physics)
Revision Note
Threshold Frequency
The photoelectric effect is the phenomenon in which electrons are emitted from the surface of a metal upon the absorption of electromagnetic radiation
Electrons removed from a metal in this manner are known as photoelectrons
The photoelectric effect provides important evidence that light behaves as a particle
Light is quantised or carried in discrete packets
This is shown by the fact each electron can absorb only a single photon
This means only the frequencies of light above a threshold frequency will emit a photoelectron
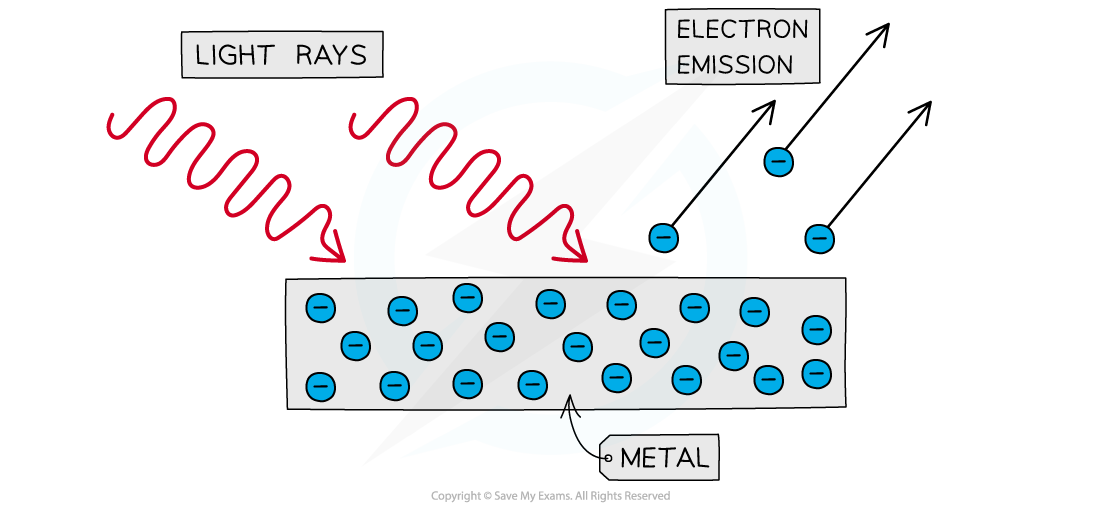
The photoelectric effect: photoelectrons are emitted from the surface of a metal when light shines on to it
Threshold Frequency & Wavelength
The threshold frequency is defined as:
The minimum frequency of incident electromagnetic radiation required to remove a photoelectron from the surface of a metal
The threshold wavelength, related to threshold frequency by the wave equation, is defined as:
The longest wavelength of incident electromagnetic radiation that would remove a photoelectron from the surface of a metal
Frequency and wavelength are related by the equation:
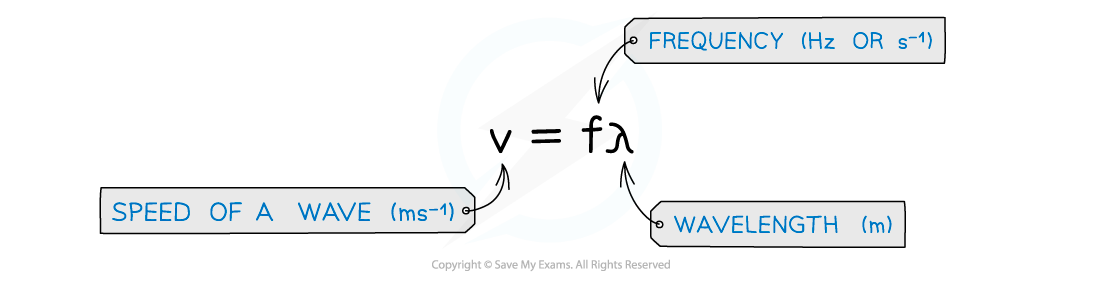
Since photons are particles of light, v = c (speed of light)
Threshold frequency and wavelength are properties of a material, and vary from metal to metal
Threshold frequencies and wavelengths for different metals
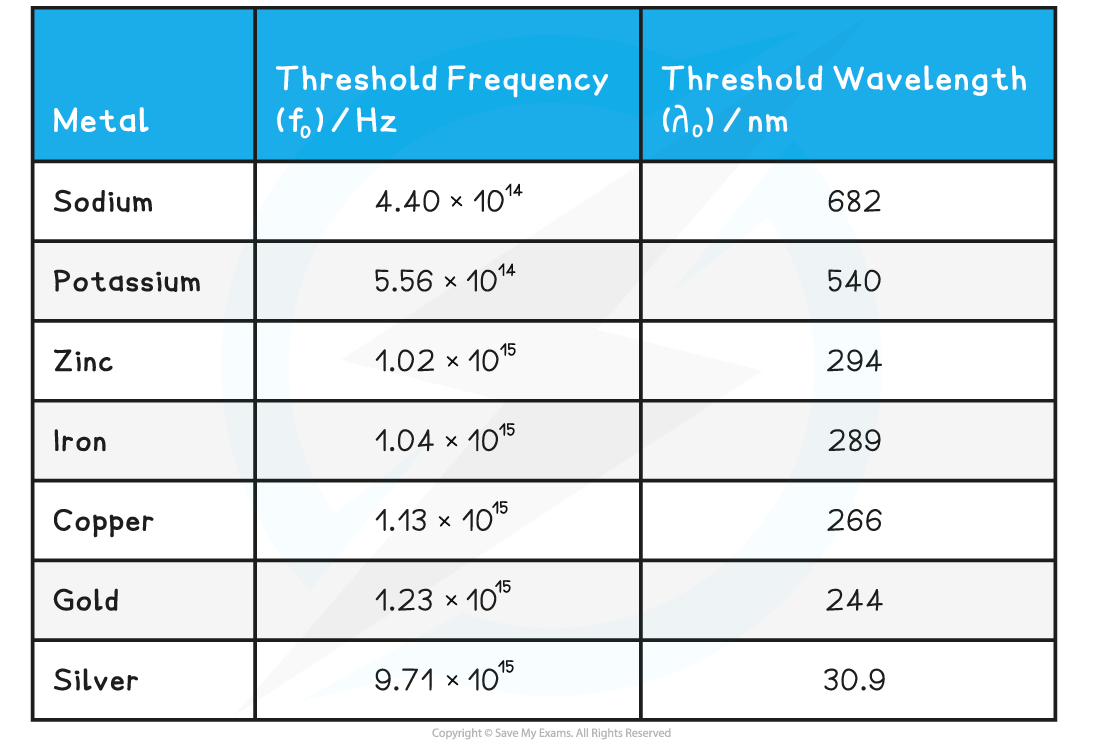
Examiner Tips and Tricks
A useful analogy for threshold frequency is a fairground coconut shy:
One person is throwing table tennis balls at the coconuts, and another person has a pistol
No matter how many of the table tennis balls are thrown at the coconut it will still stay firmly in place – this represents the low frequency photons
However, a single shot from the pistol will knock off the coconut immediately – this represents the high frequency photons
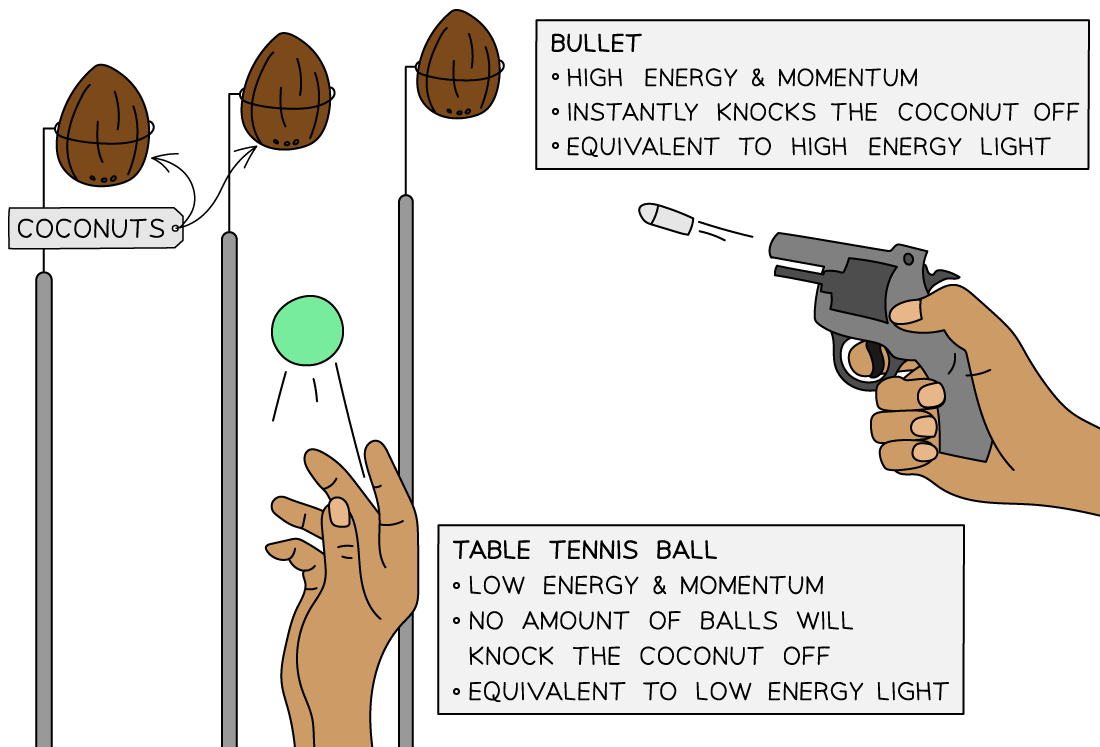
The Work Function
The work function Φ, or threshold energy, of a material, is defined as:
The minimum energy required to release a photoelectron from the surface of a metal
Consider the electrons in a metal as trapped inside an ‘energy well’ where the energy between the surface and the top of the well is equal to the work function Φ
A single electron absorbs one photon
Therefore, an electron can only escape from the surface of the metal if it absorbs a photon which has an energy equal to Φ or higher
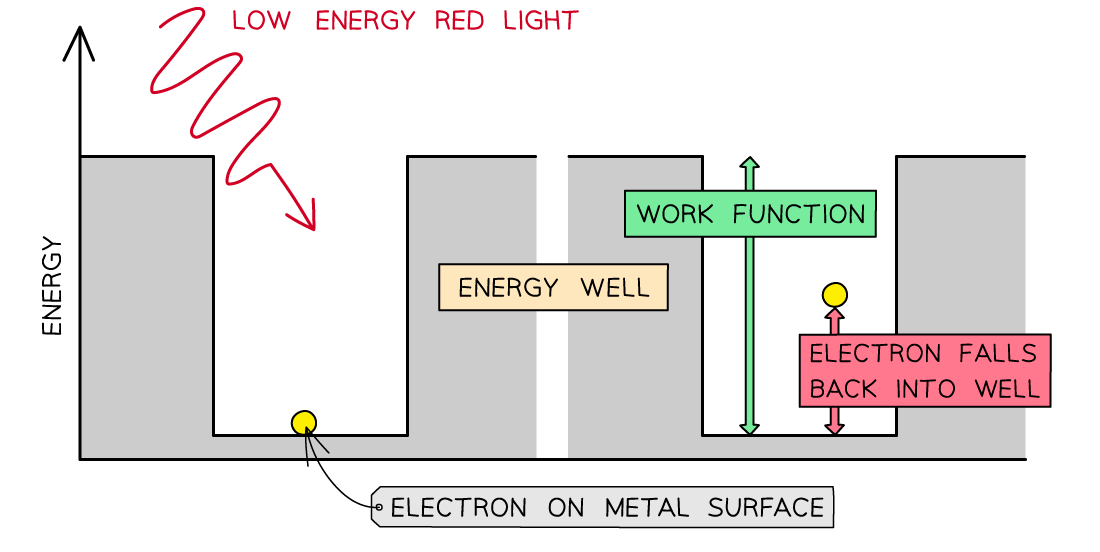
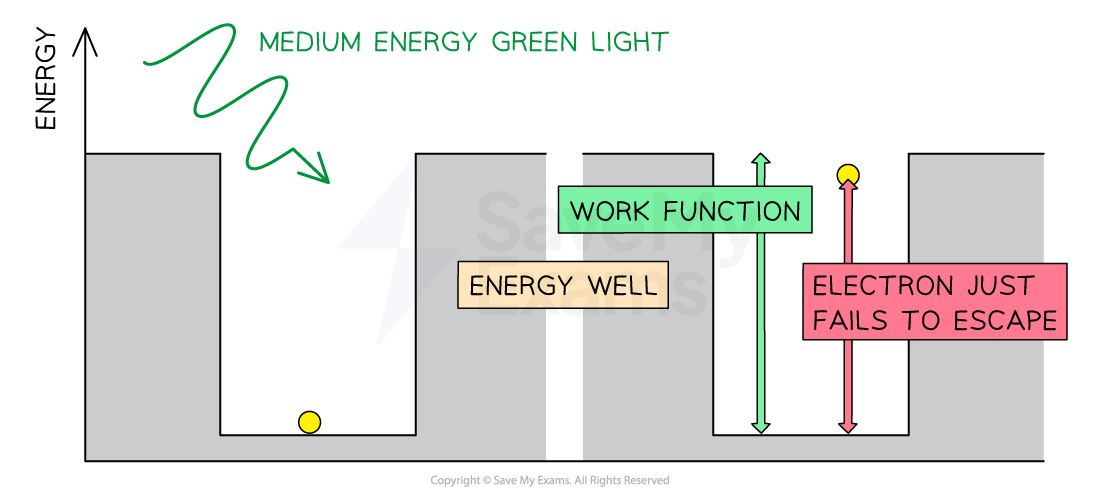
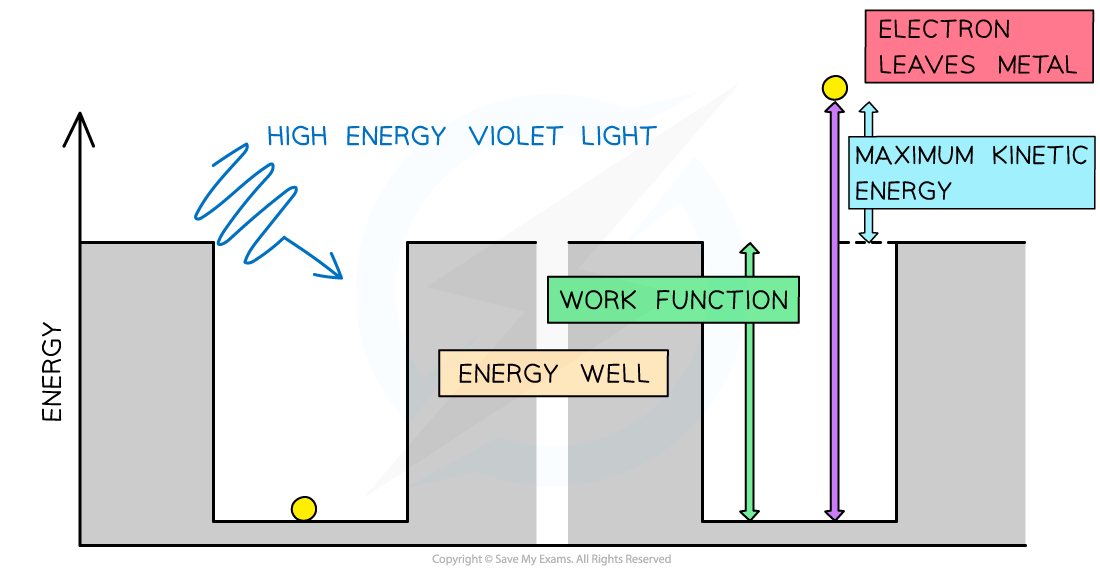
In the photoelectric effect, a single photon may cause a surface electron to be released if it has sufficient energy
Different metals have different threshold frequencies and hence different work functions
Using the well analogy:
A more tightly bound electron requires more energy to reach the top of the well
A less tightly bound electron requires less energy to reach the top of the well
Alkali metals, such as sodium and potassium, have threshold frequencies in the visible light region
This is because the attractive forces between the surface electrons and positive metal ions are relatively weak
Transition metals, such as zinc and iron, have threshold frequencies in the ultraviolet region
This is because the attractive forces between the surface electrons and positive metal ions are much stronger
Stopping Potential
Stopping potential, Vs, is defined as:
The potential difference required to stop photoelectron emission from occurring
The photons arriving at the metal plate cause photoelectrons to be emitted
This is called the emitter plate
The electrons that cross the gap are collected at the other metal plate
This is called the collector plate
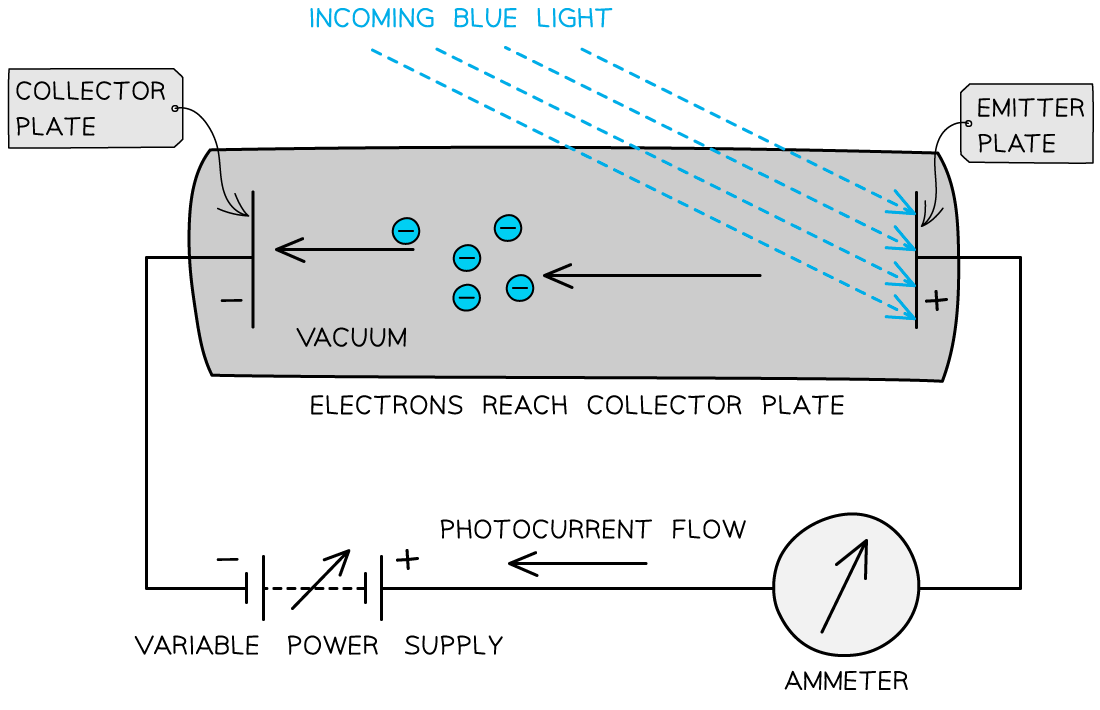
This set up can be used to determine the maximum kinetic energy of the emitted photoelectrons
The flow of electrons across the gap results in an electromotive force (e.m.f.) between the plates that causes a current to flow around the rest of the circuit
Effectively, it becomes a photoelectric cell producing a photoelectric current
If the e.m.f. of the variable power supply is initially zero, the circuit operates only on the photoelectric current
As the supply is turned up, the emitter plate becomes more positive (because it is connected to the positive terminal of the supply)
As a result, electrons leaving the emitter plate are attracted back towards it
This is because the potential difference (p.d.) across the tube opposes the motion of the electrons between the plates
If any electrons escape with enough kinetic energy, they can overcome this attraction and cross to the collector plate
And if they don't have enough energy, they can't cross the gap
By increasing the e.m.f. of the supply, eventually, a p.d. will be reached at which no electrons can cross the gap – this is the stopping potential, Vs
At this point, the energy needed to cross the gap is equal to the maximum kinetic energy
of the electrons
Therefore, the maximum kinetic energy of the photoelectrons is:
Where:
= maximum kinetic energy of photoelectrons (i.e. surface electrons)
e = charge on an electron
Vs = stopping potential
Intensity and Stopping Potential
Increasing the intensity of the incident radiation on the plate increases
The number of photons incident on the metal plate
The number of photoelectrons emitted from the plate, i.e. the photoelectric current
For a given potential difference, increasing the intensity increases the photoelectric current but the stopping potential remains the same
This shows that the intensity does not affect the kinetic energy of the photoelectrons
The maximum kinetic energy of the photons (and photoelectrons) depends only on
The frequency (or wavelength) of the incident photons
The work function of the metal
However, if the frequency or wavelength is changed whilst keeping the intensity constant, the photoelectric current will not be constant
For example, increasing the frequency of the incident radiation whilst keeping the intensity constant will cause the photoelectric current to decrease. This is because:
Increasing the frequency of a source means the energy of each photon increases
Keeping intensity the same means the energy transferred per unit area in a given time is constant
So, a higher frequency source must emit fewer photons per unit area in a given time than a lower frequency source (of the same intensity)
If there are fewer photons incident on a given area each second, the number of electrons emitted each second must decrease
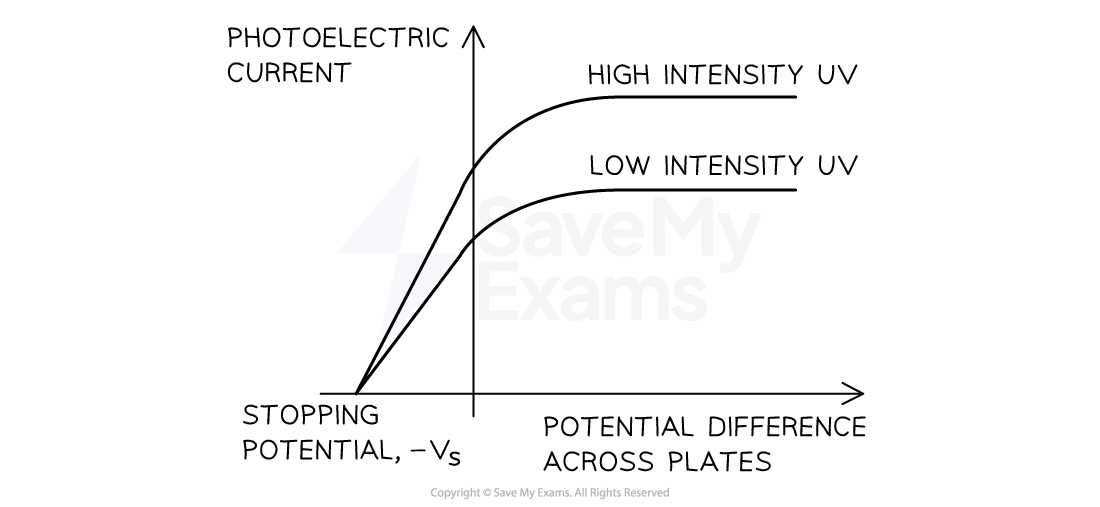
The stopping potential remains constant even at different intensities, which shows that intensity does not affect the kinetic energy of the photoelectrons
Examiner Tips and Tricks
It is important to note that the stopping voltage actually holds a negative value, but since we use it to determine the maximum kinetic energy of the emitted electrons, its sign is not important in calculations, it's acceptable to just quote its magnitude.
You've read 0 of your 5 free revision notes this week
Sign up now. It’s free!
Did this page help you?