Uses of Recombinant DNA Technology (AQA A Level Biology)
Revision Note
Written by: Lára Marie McIvor
Reviewed by: Lucy Kirkham
Interpret & Evaluate Data about the Uses of Recombinant DNA Technology
DNA that has been altered by introducing nucleotides from another source is called recombinant DNA (rDNA)
If the organism contains nucleotides from a different species it is called a transgenic organism
Any organism that has introduced genetic material is a genetically modified organism (GMO)
Recombinant DNA has been used to produce recombinant proteins (RP), thus recombinant proteins are manipulated forms of the original protein
Producing recombinant proteins
Recombinant proteins are generated using microorganisms such as bacteria, yeast, or animal cells in culture. They are used for research purposes and for treatments (eg. diabetes, cancer, infectious diseases, haemophilia)
Most recombinant human proteins are produced using eukaryotic cells (eg. yeast, or animal cells in culture) rather than using prokaryotic cells, as these cells will carry out the post-translational modification (due to the presence of Golgi Apparatus and/or enzymes) that is required to produce a suitable human protein
The advantages of genetic engineering organisms to produce recombinant human proteins are:
More cost-effective to produce large volumes (i.e. there is an unlimited availability)
Simpler (with regards to using prokaryotic cells)
Faster to produce many proteins
Reliable supply available
The proteins are engineered to be identical to human proteins or have modifications that are beneficial
It can solve the issue for people who have moral or ethical or religious concerns against using cow or pork produced proteins
Insulin
In 1982, insulin was the first recombinant human protein to be approved for use in diabetes treatment
Bacteria plasmids are modified to include the human insulin gene
Restriction endonucleases are used to cut open plasmids and DNA ligase is used to splice the plasmid and human DNA together
These recombinant plasmids are then inserted into Escherichia coli by transformation (bath of calcium ions and then heat or electric shock)
Once the transgenic bacteria are identified (by the markers), they are isolated, purified and placed into fermenters that provide optimal conditions
The transgenic bacteria multiply by binary fission, and express the human protein - insulin, which is eventually extracted and purified
The advantages for scientists to use recombinant insulin are:
It is identical to human insulin, unless modified to have different properties (eg. act faster, which is useful for taking immediately after a meal or to act more slowly)
There is a reliable supply available to meet demand (no need to depend on availability of meat stock)
Fewer ethical, moral or religious concerns (proteins are not extracted from cows or pigs)
Fewer rejection problems or side effects or allergic reactions
Cheaper to produce in large volumes
That it is useful for people who have animal insulin tolerance
Factor VIII
Factor VIII is a blood-clotting protein that haemophiliacs cannot produce
Kidney and ovary hamster cells have been genetically modified to produce Factor VIII
Once modified these recombinant cells are placed into a fermenter and cultured
Due to the optimal conditions in the fermenter, the hamster cells constantly express Factor VIII which can then be extracted and purified, and used as an injectable treatment for haemophilia
The advantages for scientists to use recombinant Factor VIII are:
Fewer ethical, moral or religious concerns (proteins are not extracted from human blood)
Less risk of transmitting infection (eg. HIV) or disease
Greater production rate
Gene therapy
The use of gene therapy in medicine is becoming more common
Gene therapy involves using various mechanisms to alter a person’s genetic material to treat, or cure, diseases
As scientists gain a better understanding of the human genome and therefore the location of genes that cause genetic disorders, the possibilities of gene therapy being able to replace a faulty gene, inactivate a faulty gene or insert a new gene are growing
Most gene therapies are still in the clinical trial stage because scientists are having difficulty finding delivery systems that can transfer normal alleles into a person’s cells and how to ensure the gene is correctly expressed once there
Gene therapy is being used in medicine for introducing corrected copies of genes into patients with genetic diseases (eg. cystic fibrosis, haemophilia, severe combined immunodeficiency)
Currently, all gene therapies have targeted and been tested on somatic (body)
Changes in genetic material are targeted to specific cells and so will not be inherited by future generations (as somatic gene therapy does not target the gametes)
Often the effects of changing the somatic cells are short-lived
There are two types of somatic gene therapy:
Ex vivo – the new gene is inserted via a virus vector into the cell outside the body. Blood or bone marrow cells are extracted and exposed to the virus which inserts the gene into these cells. These cells are then grown in the laboratory and returned to the person by injection into a vein
In vivo – the new gene is inserted via a vector into cells inside the body
There is the potential for new genetic material to be inserted into germ cells (cells involved in sexual reproduction eg. gametes or an early embryo)
However, this is illegal in humans as any changes made to the genetic material of these cells is potentially permanent and could therefore be inherited by future generations
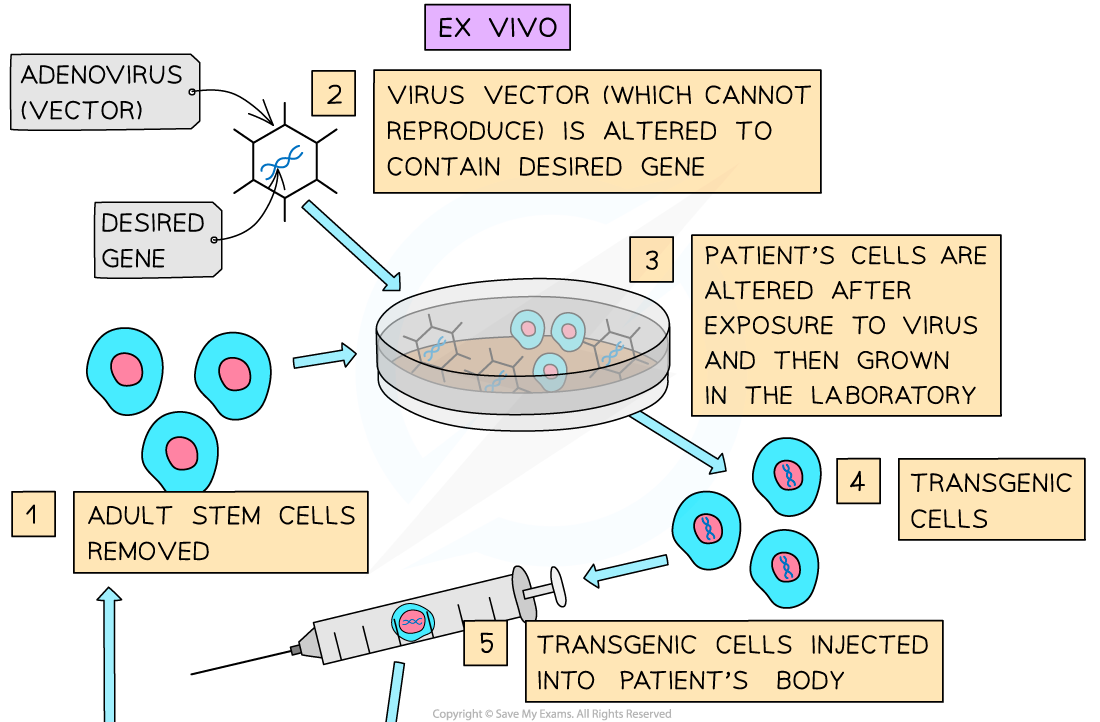
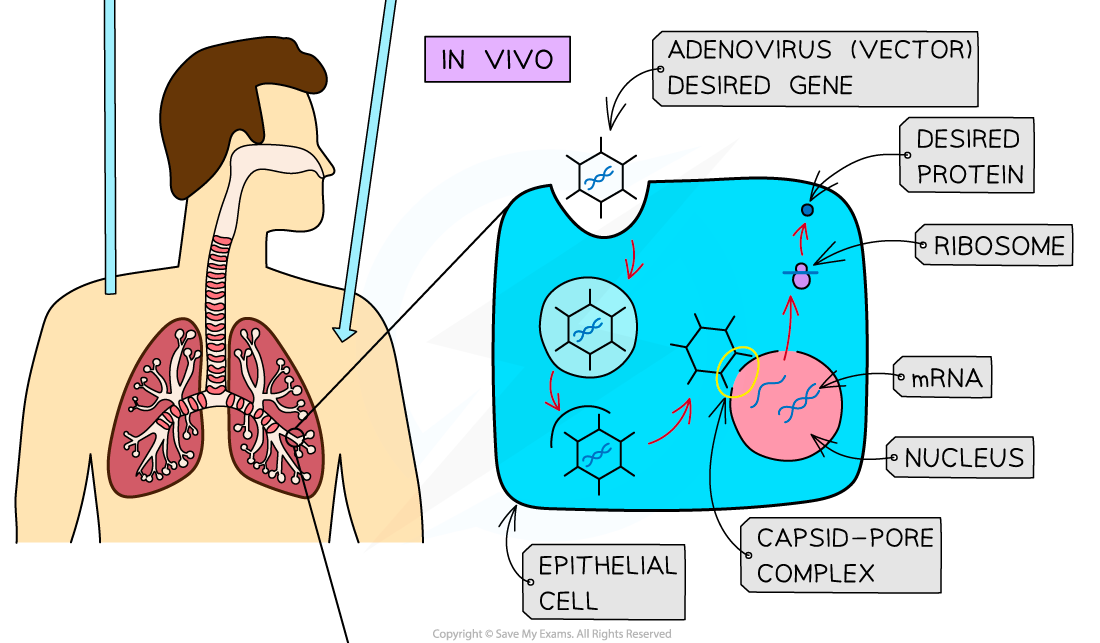
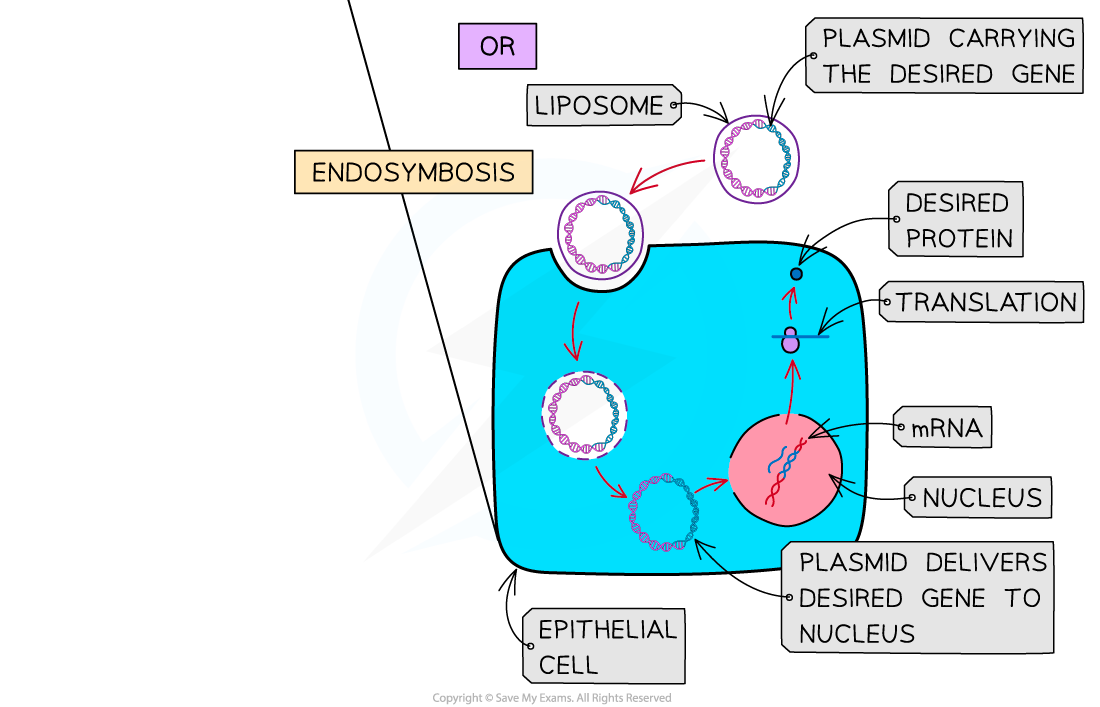
The two types of somatic gene therapy - ex vivo and in vivo. Two of the methods scientists are using to alter a patient’s genetic material to treat or cure diseases.
Severe combined immunodeficiency (SCID)
Severe combined immunodeficiency (SCID) is caused by the body's inability to produce adenosine deaminase (ADA), an enzyme that is key to the functioning of the immune system. Without this enzyme, children can die from common infections and therefore need to be kept isolated often inside plastic ‘bubbles’
To treat SCID scientists have used ex vivo somatic gene therapy. During this therapy, a virus transfers a normal allele for ADA into T-lymphocytes removed from the patient and the cells are then returned via an injection
This is not a permanent cure as the T-lymphocytes are replaced by the body over time and therefore the patient requires regular transfusions every three to five months to keep their immune systems functioning
Originally retroviruses were used as the vectors, however these viruses insert their genes randomly into a host’s genome which means they could insert the gene into another gene or into a regulatory sequence of a gene (which could result in cancer)
Initial treatments did cause cases of leukaemia in children, so researchers switched to using lentiviruses or adeno-associated viruses as vectors. Lentiviruses also randomly insert their genes into the host genome however they can be modified to not replicate, whereas adeno-associated viruses do not insert their genes into the host genome and therefore the genes are not passed onto the daughter cells when a cell divides. This is an issue with short-lived cells like lymphocytes but has not been a problem when used with longer living cells such as liver cells
Social & ethical considerations associated with gene therapy
The social and ethical considerations of using gene therapy include:
The potential for side effects that could cause death (eg. the children who were treated for SCID developed leukaemia)
Whether germline gene therapy (the alteration of genes in egg and sperm cells which results in the alteration being passed onto future generations) should be allowed – it could be a cure for a disease or it could create long-term side effects
The commercial viability for pharmaceutical companies – if it is a rare disease, the relative small number of patients may not mean that the companies will make a profit (eg. Glybera – a gene therapy for lipoprotein lipase deficiency is no longer produced as there were too few patients)
The expense of treatments as multiple injections of the genes may be required if the somatic cells are short-lived (eg. severe combined immunodeficiency). This may make the cost of gene therapy accessible to a limited number of people
The possibility that people will become less accepting of disabilities as they become less common
Who has the right to determine which genes can be altered and which cannot (eg. should people be allowed to enhance intelligence or height)
Another method of enhancing sporting performances unfairly through gene doping. This is where the genes are altered to give an unfair advantage eg. to provide a source of erythropoietin (the hormone that promotes the formation of red blood cells)
Genetic engineering in agriculture
Although plants and animals have been genetically engineered to produce proteins used in medicine, the main purpose for genetically engineering them is to meet the global demand for food
Crop plants have been genetically modified to be:
Resistant to herbicides – increases productivity / yield
Resistant to pests – increases productivity / yield
Enriched in vitamins – increases the nutritional value
Farmed animals have been genetically modified to grow faster. It is rarer for animals to be modified for food production due to ethical concerns associated with this practice
Scientists have genetically modified many organisms including bacteria (eg. to produce insulin), sheep (eg. to produce a human blood protein known as AAT), maize (eg. to be resistant to insect attacks), rice (eg. to produce β-carotene to provide vitamin A)
The benefits of using genetic engineering rather than the more traditional selective breeding techniques to solve the global demand for food are:
Organisms with the desired characteristics are produced more quickly
All organisms will contain the desired characteristic (there is no chance that recessive allele may arise in the population)
The desired characteristic may come from a different species / kingdom
GM salmon
In 2015 AquaAdventure Salmon was approved by the US Food and Drug Authority (FDA) for human consumption
This salmon has been genetically modified (GM) to grow more rapidly than non-GM salmon as a result of growth hormone being produced in the salmon throughout the year, instead of just in spring and summer. The producer therefore has a product to sell in half the time, which increases their yield
Scientists combined a growth hormone gene from a chinook salmon with the promoter gene from an ocean pout, a cold-water fish. The ocean pout fish can grow in near-freezing waters, thus the promoter gene ensured the growth hormone was continually being expressed
To prevent the GM salmon from reproducing in the wild, all the salmon are female and sterile
Insect resistance in cotton
Cotton has been genetically modified with a gene for the Bt toxin, which is taken from the bacterium Bacillus thuringiensis
Cotton plants modified with the Bt toxin gene produce their own insecticide
When an insect ingests parts of the cotton plant, the alkaline conditions in their guts activate the toxin (the toxin is harmless to vertebrates as their stomach is highly acidic), killing the insect
Different strains of thuringiensis produce different toxins which are toxic to different insect species
Insect populations have developed resistance to the genes for Bt toxin, reducing its effectiveness as a means of protecting crops
Social and ethical implications associated with GMOs in agriculture
The genetic modification of microorganisms for the production of medicines, antibiotics and enzymes raises little debate compared to the use of genetically modified organisms (GMOs) for food production
The use of GMOs in food production has been proposed as a solution to feeding the increasing world population, the decreasing arable land and decreasing the impact on the environment, however concerns such as the development of resistance in insects and weeds and costs of seeds have meant that countries are not allowing GMOs to be grown
The solution could be integrated pest-management systems that could help avoid the development of resistance and increased population of secondary pests
The ethical implications of using GMOs in food production are:
The lack of long-term research on the effects on human health – should GM food be consumed if it is unknown whether it will cause allergies or be toxic over time (although there has been no evidence to suggest this would occur to date)
Making choices for others:
That without appropriate labelling the consumer cannot make an informed decision about the consumption of GM foods
As the pollen from the GM crop may contaminate nearby non-GM crops that have been certified as organic
By reducing the biodiversity for future generations
The social implications of growing GMOs for food evolve around whether the crops are safe for human consumption and for the surrounding environment
The possible implications are:
The GM crops may become weeds or invade the natural habitats bordering the farmland
The development of resistance for the introduced genes in the wild relative populations
Potential ecological effects (e.g. harm to non-targeted species like the Monarch butterflies)
Cost to farmers (new seed needs to purchase each year)
The ability to provide enriched foods to those suffering from deficiencies (eg. Golden Rice) and therefore decrease diseases
Reduced impact on the environment due to there being less need to spray pesticides (eg. less beneficial insects being harmed)
Reduction in biodiversity which could affect food webs
The herbicides that are used on the GM crops could leave toxic residues
Last updated:
You've read 0 of your 5 free revision notes this week
Sign up now. It’s free!
Did this page help you?